Why HCN Doesn't Dissociate: Weak Acid Explained
Hydrogen cyanide (HCN), a compound famously explored in chemistry textbooks, exhibits weak acid properties, a characteristic stemming from its limited ionization in aqueous solutions. The acid dissociation constant, Ka, for HCN, is significantly low, around 6.2 x 10-10, indicating that only a small fraction of HCN molecules dissociate into hydrogen ions (H+) and cyanide ions (CN-). This behavior contrasts sharply with strong acids like hydrochloric acid (HCl), which completely dissociate in water; the central question of why does HCN not dissociation fully arises from this comparison. The low dissociation rate is attributed to the strong covalent bond between hydrogen and cyanide, influenced by Linus Pauling's electronegativity principles. This foundational understanding is critical in environmental contexts, where HCN's presence, even in low concentrations, demands precise monitoring to mitigate potential toxicity as discussed by the Environmental Protection Agency (EPA).
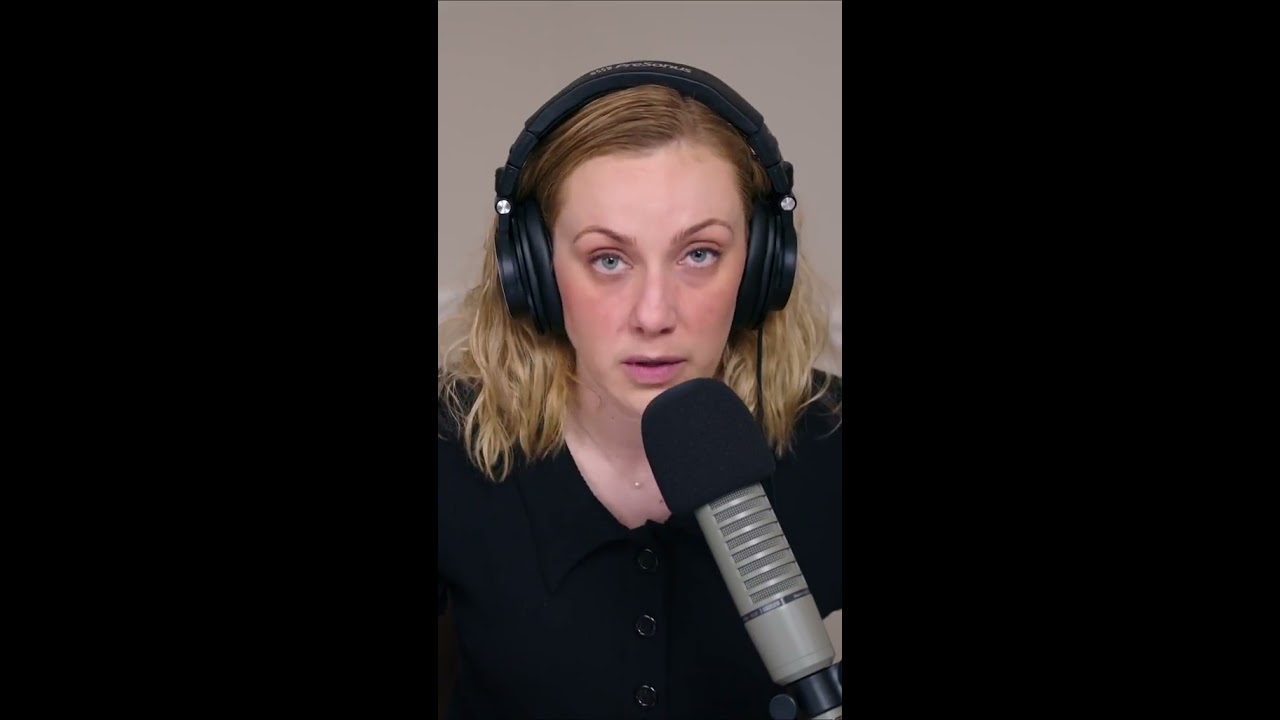
Image taken from the YouTube channel Kati Morton , from the video titled What DISSOCIATION Really Is! Have YOU experienced this? Let me know below. .
Hydrogen Cyanide (HCN): An Overview of its Weak Acid Properties
Hydrogen Cyanide (HCN), a chemical compound with the formula HCN, is a volatile, colorless, and extremely poisonous liquid. It's a compound that demands respect and a thorough understanding due to its widespread presence and varied roles in chemical processes.
While infamous for its toxicity, HCN is also a crucial intermediate in various industrial processes, including the production of polymers and other synthetic materials. Its presence extends beyond industrial applications, playing a role in biological systems and environmental chemistry. Understanding its chemical properties is therefore paramount.
Defining a Weak Acid
At the core of understanding HCN lies its classification as a weak acid.
Unlike strong acids, which fully dissociate into ions in aqueous solutions, weak acids only partially dissociate. This means that when HCN is dissolved in water, it establishes an equilibrium between the undissociated HCN molecules and its constituent ions: the hydrogen ion (H+) and the cyanide ion (CN-).
The degree of dissociation is quantified by the acid dissociation constant (Ka), a value that reflects the acid's tendency to donate a proton (H+). The higher the Ka value, the stronger the acid. For HCN, the Ka value is relatively low, indicating its weak acid nature.
The Significance of Understanding HCN's Acidity
Understanding the acidic properties of HCN is essential for several reasons.
Firstly, it dictates its reactivity in chemical reactions. The partial dissociation of HCN affects its ability to act as a proton donor, influencing its interactions with other chemical species.
Secondly, its acidity influences its behavior in different environments. Factors such as pH and temperature can shift the equilibrium of HCN dissociation, affecting its concentration and reactivity.
Scope of Analysis
This analysis will delve into the properties of HCN as a weak acid, exploring its dissociation dynamics, quantifying its acidity using Ka and pKa values, and examining the factors that influence its behavior in solution.
By understanding these properties, we can better appreciate the role of HCN in various chemical contexts and its potential impact on our environment and health.
Understanding Acidity: Acid-Base Theories and HCN's Role
Hydrogen Cyanide (HCN) is frequently categorized as a weak acid, but to truly appreciate this classification, a review of fundamental acid-base theories is essential. These theories provide the framework for understanding how HCN interacts with other substances and behaves in solution. This section will delve into these theories, specifically focusing on the Brønsted-Lowry definition of acids and bases, and how HCN fits into this model. We will also contrast HCN's behavior with that of strong acids, thereby elucidating the key differences that define its weak acid character.
Brønsted-Lowry Acid-Base Theory and HCN
The Brønsted-Lowry theory defines an acid as a proton (H+) donor and a base as a proton acceptor. This definition is crucial for understanding HCN's acidic properties. In aqueous solutions, HCN donates a proton to water, forming the cyanide ion (CN-) and hydronium ion (H3O+).
The reaction can be represented as follows:
HCN(aq) + H2O(l) ⇌ H3O+(aq) + CN-(aq)
Here, HCN acts as the Brønsted-Lowry acid by donating a proton to water, which acts as the Brønsted-Lowry base. The cyanide ion (CN-) is the conjugate base of HCN, and the hydronium ion (H3O+) is the conjugate acid of water. This equilibrium reaction is fundamental to understanding HCN's behavior in solution.
HCN as a Proton Donor
HCN's ability to donate a proton is what classifies it as an acid within the Brønsted-Lowry framework. However, it's the extent to which it donates protons that distinguishes it as a weak acid. Unlike strong acids that completely dissociate in water, HCN only partially dissociates. This partial dissociation is a key characteristic that sets it apart from its stronger counterparts.
Contrasting HCN with Strong Acids: A Matter of Degree
To fully grasp the concept of HCN as a weak acid, it is imperative to contrast its behavior with that of strong acids. Consider hydrochloric acid (HCl), a quintessential strong acid. When HCl is dissolved in water, it completely dissociates into hydrogen ions (H+) and chloride ions (Cl-):
HCl(aq) → H+(aq) + Cl-(aq)
This complete dissociation means that, for all practical purposes, no undissociated HCl molecules remain in solution. The reaction proceeds essentially to completion.
Differences in Dissociation
The contrast between HCN and HCl lies in the extent of dissociation. While HCl undergoes complete dissociation, HCN only partially dissociates. This means that in a solution of HCN, there will be a significant amount of undissociated HCN molecules present alongside the cyanide and hydronium ions.
This difference in dissociation has profound implications for the acidity of the solution. A strong acid like HCl produces a high concentration of hydrogen ions (H+), resulting in a low pH and high acidity. In contrast, a weak acid like HCN produces a much lower concentration of H+ ions, leading to a higher pH (though still acidic) and a correspondingly lower acidity.
The partial dissociation of HCN is due to the relatively strong bond between the hydrogen and carbon atoms in the molecule. This bond requires more energy to break compared to the bond in a strong acid like HCl, hence the difference in dissociation behavior.
Ultimately, understanding the Brønsted-Lowry acid-base theory and contrasting HCN's behavior with that of strong acids is crucial for comprehending its classification as a weak acid. The partial dissociation of HCN, compared to the complete dissociation of strong acids, is the defining characteristic that sets it apart.
Dissociation Dynamics: How HCN Behaves in Aqueous Solutions
Hydrogen Cyanide (HCN) is frequently categorized as a weak acid, but to truly appreciate this classification, a review of fundamental acid-base theories is essential. These theories provide the framework for understanding how HCN interacts with other substances and behaves in solution. This section will delve into the dynamics of HCN dissociation in aqueous solutions, exploring the underlying mechanisms and factors that govern its behavior.
Understanding Dissociation: The Breakdown of HCN in Water
When HCN is introduced into water, it undergoes a process known as dissociation. Dissociation refers to the separation of a compound into its constituent ions when dissolved in a solvent, in this case, water.
For HCN, this involves the breaking of the covalent bond between the hydrogen and cyanide atoms. This leads to the formation of hydrogen ions (H+) and cyanide ions (CN-).
The process can be represented by the following equilibrium reaction:
HCN(aq) ⇌ H+(aq) + CN-(aq)
It's important to note that, unlike strong acids which dissociate almost completely, HCN only dissociates partially. This is a key characteristic of weak acids and contributes to its distinct chemical properties.
Establishing Equilibrium: A State of Dynamic Balance
The dissociation of HCN in water doesn't proceed to completion; instead, it reaches a state of equilibrium. Chemical equilibrium is a dynamic state where the rate of the forward reaction (dissociation of HCN) equals the rate of the reverse reaction (recombination of H+ and CN- to form HCN).
At equilibrium, the concentrations of HCN, H+, and CN- remain constant over time, even though the forward and reverse reactions continue to occur.
The position of the equilibrium – that is, the relative concentrations of reactants and products – determines the extent of dissociation. For HCN, the equilibrium lies far to the left, indicating that only a small fraction of HCN molecules dissociate into ions.
The Role of the Cyanide Ion (CN-): The Conjugate Base
The cyanide ion (CN-) plays a crucial role in the equilibrium of HCN dissociation as the conjugate base of the weak acid, HCN.
The conjugate base is what remains of the acid after it has donated a proton (H+). In this case, when HCN donates a proton, it forms the cyanide ion.
The strength of the conjugate base is inversely related to the strength of the acid. Since HCN is a weak acid, its conjugate base, CN-, is relatively strong.
This means that the cyanide ion has a significant affinity for protons and can readily accept them to reform HCN.
This tendency of CN- to accept protons influences the equilibrium of the reaction, shifting it back towards the undissociated HCN. The influence of the conjugate base (CN-) is a critical factor in understanding the behavior of HCN in aqueous solutions, reinforcing its classification as a weak acid.
Quantifying Acidity: Ka and pKa Values for HCN
Hydrogen Cyanide (HCN) is frequently categorized as a weak acid, but to truly appreciate this classification, a review of fundamental acid-base theories is essential. These theories provide the framework for understanding how HCN interacts with other substances and behaves in solution. This brings us to the quantitative aspects of acidity, specifically, the Acid Dissociation Constant (Ka) and its logarithmic counterpart, pKa. These values provide a precise measure of HCN's acidic strength and offer insights into its behavior in aqueous solutions.
The Acid Dissociation Constant (Ka): A Quantitative Measure of Acid Strength
The Acid Dissociation Constant, represented as Ka, is a numerical expression of the extent to which an acid dissociates in water. In simpler terms, it quantifies how readily an acid donates protons (H+) to form its conjugate base.
For HCN, the dissociation reaction in water can be represented as follows:
HCN(aq) + H2O(l) ⇌ H3O+(aq) + CN-(aq)
The Ka for this reaction is defined as:
Ka = [H3O+][CN-] / [HCN]
Where the square brackets denote the equilibrium concentrations of the respective species.
Relevance of Ka to HCN
The Ka value for HCN is approximately 6.2 x 10-10 at 25°C. This small value indicates that only a tiny fraction of HCN molecules dissociate into ions in water.
This directly reflects HCN's weak acid nature; a higher Ka would signify a stronger acid with a greater propensity to donate protons. The very low Ka value for HCN, thus, serves as quantitative evidence of its classification as a weak acid.
The small degree of dissociation underscores that, at equilibrium, the concentration of undissociated HCN molecules is significantly higher than the concentrations of hydronium ions (H3O+) and cyanide ions (CN-).
This has profound implications for the chemical behavior of HCN, influencing its reactivity and interactions with other chemical species.
pKa: An Alternative Measure of Acidity
While Ka provides a direct measure of acid strength, pKa offers a more convenient and intuitive scale for comparing the acidity of different substances.
pKa is defined as the negative base-10 logarithm of Ka:
pKa = -log10(Ka)
This logarithmic transformation converts the typically small and unwieldy Ka values into more manageable numbers. A lower pKa indicates a stronger acid, while a higher pKa indicates a weaker acid.
Significance of the High pKa Value of HCN
Given that the Ka of HCN is approximately 6.2 x 10-10, its pKa value can be calculated as follows:
pKa = -log10(6.2 x 10-10) ≈ 9.21
The pKa value of 9.21 for HCN further solidifies its classification as a weak acid. In general, acids with pKa values greater than approximately 2 are considered weak acids.
For comparison, strong acids like hydrochloric acid (HCl) have pKa values that are negative (e.g., -6 to -8), indicating their significantly greater acidity. The high pKa of HCN, therefore, is a critical parameter in understanding its chemical properties and behavior. It emphasizes that, under typical conditions, HCN exists primarily in its undissociated form, influencing its reactivity and interactions in chemical systems.
Influencing Factors: Exploring What Affects HCN Acidity
Hydrogen Cyanide (HCN) is frequently categorized as a weak acid, but to truly appreciate this classification, a review of fundamental acid-base theories is essential. These theories provide the framework for understanding how HCN interacts with other substances and behaves in solution. This brings us to the critical examination of factors that influence HCN's acidity, providing a more nuanced understanding of its chemical behavior.
Electronegativity and Bond Polarity in HCN
The acidity of HCN, or any acid for that matter, is not an inherent, immutable property. Instead, it's a characteristic influenced by a confluence of factors at the molecular level. One of the most significant is electronegativity, the measure of an atom's ability to attract electrons in a chemical bond.
In HCN, nitrogen is significantly more electronegative than both carbon and hydrogen. This difference in electronegativity is the foundation of the molecule's polarity.
Nitrogen's stronger pull on electrons results in a polar covalent bond between the carbon and nitrogen atoms.
Understanding the Dipole Moment
This unequal sharing creates a dipole moment within the molecule, with the nitrogen atom carrying a partial negative charge (δ-) and the carbon atom bearing a partial positive charge (δ+). The hydrogen atom, bound to the carbon, becomes more susceptible to ionization.
This inherent polarity weakens the H-C bond, making it easier for HCN to donate a proton (H+) and act as an acid.
However, this effect is not strong enough to make HCN a strong acid. The hydrogen atom remains relatively tightly bound, contributing to HCN's classification as a weak acid.
The H-C Bond and Its Impact
The H-C bond is the focal point of HCN's acidic behavior. The extent to which this bond is polarized directly influences the ease with which the hydrogen can be released as a proton.
If the bond were exceptionally weak, HCN would readily donate protons, behaving as a strong acid. Conversely, a strong, non-polar H-C bond would render HCN virtually non-acidic. The reality lies somewhere in between, resulting in HCN's characteristic weak acidity.
Le Chatelier's Principle and Equilibrium Shifts
Beyond the intrinsic molecular properties of HCN, external factors also play a crucial role in determining its acidity in solution. These influences are best understood through Le Chatelier's Principle, which states that if a change of condition is applied to a system in equilibrium, the system will shift in a direction that relieves the stress.
In the context of HCN, this principle dictates how the equilibrium between HCN and its dissociated ions (H+ and CN-) responds to changes in temperature, pressure, or concentration of reactants and products.
Temperature Effects
Increasing the temperature of an HCN solution generally favors the endothermic direction of the reaction. In the case of HCN dissociation, the reaction is slightly endothermic.
This means that higher temperatures will encourage a greater degree of dissociation, increasing the concentration of H+ ions and thus raising the acidity of the solution.
The Common Ion Effect
Adding a common ion, such as cyanide (CN-), to the solution will shift the equilibrium to the left, according to Le Chatelier's Principle.
This reduces the dissociation of HCN and decreases the concentration of H+ ions, thus lowering the acidity. This is a prime example of the common ion effect, a critical concept in understanding acid-base equilibria.
pH Changes in HCN Solutions
The pH of the solution directly influences the equilibrium of HCN dissociation. Adding a strong acid will increase the concentration of H+ ions, shifting the equilibrium to the left and suppressing HCN dissociation. Conversely, adding a base will neutralize H+ ions, encouraging HCN to dissociate further to replenish them, thereby increasing acidity to a certain extent, until equilibrium is re-established.
These external influences demonstrate that acidity is not merely an intrinsic property but also a dynamic characteristic susceptible to environmental conditions. Understanding these factors provides a more complete and nuanced perspective on the behavior of HCN as a weak acid.
HCN in Action: Solution Chemistry and Reaction Rates
Hydrogen Cyanide (HCN) is frequently categorized as a weak acid, but to truly appreciate this classification, a review of fundamental acid-base theories is essential. These theories provide the framework for understanding how HCN interacts with other substances and behaves in solution. This section delves into the practical implications of HCN's weak acidity, exploring its behavior in various solutions and comparing its reaction kinetics to those of strong acids.
HCN in Aqueous Solutions
HCN's behavior in aqueous solutions is fundamentally governed by its weak acid nature. Unlike strong acids, which completely dissociate into ions, HCN only partially dissociates when dissolved in water. This partial dissociation establishes an equilibrium between the undissociated HCN molecules and the hydrogen (H+) and cyanide (CN-) ions.
The concentration of these ions dictates the solution's acidity, and since HCN's dissociation is limited, the resulting solution is less acidic compared to a solution of a strong acid at the same concentration. This equilibrium is crucial in understanding the reactivity of HCN in various chemical processes.
Reactivity with Metals and Bases
HCN's behavior isn't confined to simple aqueous solutions; it engages in a variety of reactions with other chemical species. Notably, HCN can react with certain metals to form cyanides, which are often highly toxic compounds. These reactions are significant in industrial chemistry, where cyanides are used in processes like metal extraction.
HCN also reacts with bases, undergoing neutralization reactions to form cyanide salts. These reactions underscore HCN's role as a proton donor, as it readily donates a proton to the base, resulting in the formation of water and a cyanide salt. The extent of this reaction is dictated by the strength of the base and the concentration of HCN.
Chemical Kinetics: A Tale of Two Acids
The rate at which HCN reacts, or its chemical kinetics, is a critical aspect of its behavior. Compared to strong acids like hydrochloric acid (HCl), HCN reacts much more slowly. This difference in reaction rate is a direct consequence of HCN's weak acidity and limited dissociation.
Dissociation Rate vs. Reaction Rate
Strong acids dissociate virtually instantaneously in water, leading to a rapid release of hydrogen ions. This rapid increase in H+ ions allows for fast reactions with other species. HCN, however, dissociates slowly, limiting the availability of H+ ions and consequently slowing down its reactions.
The reaction rate is also influenced by activation energy and collision frequency. However, the inherent slowness of HCN's dissociation is a dominant factor that explains the observed kinetic differences between HCN and strong acids.
The Impact on Reaction Mechanisms
The slower reaction rate of HCN has significant implications for reaction mechanisms. Reactions involving HCN often proceed through different pathways or require catalysts to accelerate the process. This is because the slow release of cyanide ions from HCN can be a rate-determining step in many chemical reactions.
In contrast, reactions with strong acids tend to proceed more directly due to the immediate availability of hydrogen ions. Understanding these kinetic differences is crucial in designing and controlling chemical processes involving HCN.
Video: Why HCN Doesn't Dissociate: Weak Acid Explained
FAQs: Why HCN Doesn't Dissociate
Why is HCN considered a weak acid?
HCN is a weak acid because it doesn't fully dissociate into H+ and CN- ions when dissolved in water. Only a small fraction of HCN molecules break apart, meaning it doesn't readily donate protons (H+). This limited dissociation is the hallmark of a weak acid.
What does "dissociation" mean in this context?
Dissociation refers to the process where a compound breaks apart into ions when dissolved in a solvent like water. Strong acids dissociate completely, whereas weak acids, like HCN, only partially dissociate. This incomplete breaking apart is why does hcn not dissociation fully in solution.
What factors contribute to HCN's weak acidity?
The weak acidity of HCN is primarily due to the strong bond between hydrogen (H) and carbon (C). This strong bond makes it difficult to release the hydrogen ion (H+), which is essential for acidic behavior. Therefore, why does hcn not dissociation comes down to the strength of the H-C bond and its resistance to breaking.
How does the dissociation constant (Ka) relate to HCN's weakness?
The dissociation constant, Ka, is a quantitative measure of an acid's strength. HCN has a very small Ka value, indicating that the equilibrium favors undissociated HCN molecules rather than H+ and CN- ions. A small Ka directly reflects why does hcn not dissociation to a great extent.
So, next time you're thinking about acids and bases, remember that HCN's reluctance to give up its proton is a prime example of a weak acid in action. The interplay of electronegativity and bond strength ultimately explains why does HCN not dissociate readily, making it a fascinating case study in chemical equilibrium. Keep exploring the nuances of chemistry – there's always something interesting to uncover!