Enveloped Virus: Unveiling the Hidden Threat (You Must Know)
Enveloped viruses, a significant area of study for the Centers for Disease Control and Prevention (CDC), possess a distinct lipid membrane, which is an attribute influencing their infectivity. The viral load associated with enveloped viruses often dictates the severity of infection, a critical factor considered during antiviral drug development. Understanding the mechanisms by which the enveloped virus interacts with host cells remains paramount in mitigating potential public health crises.
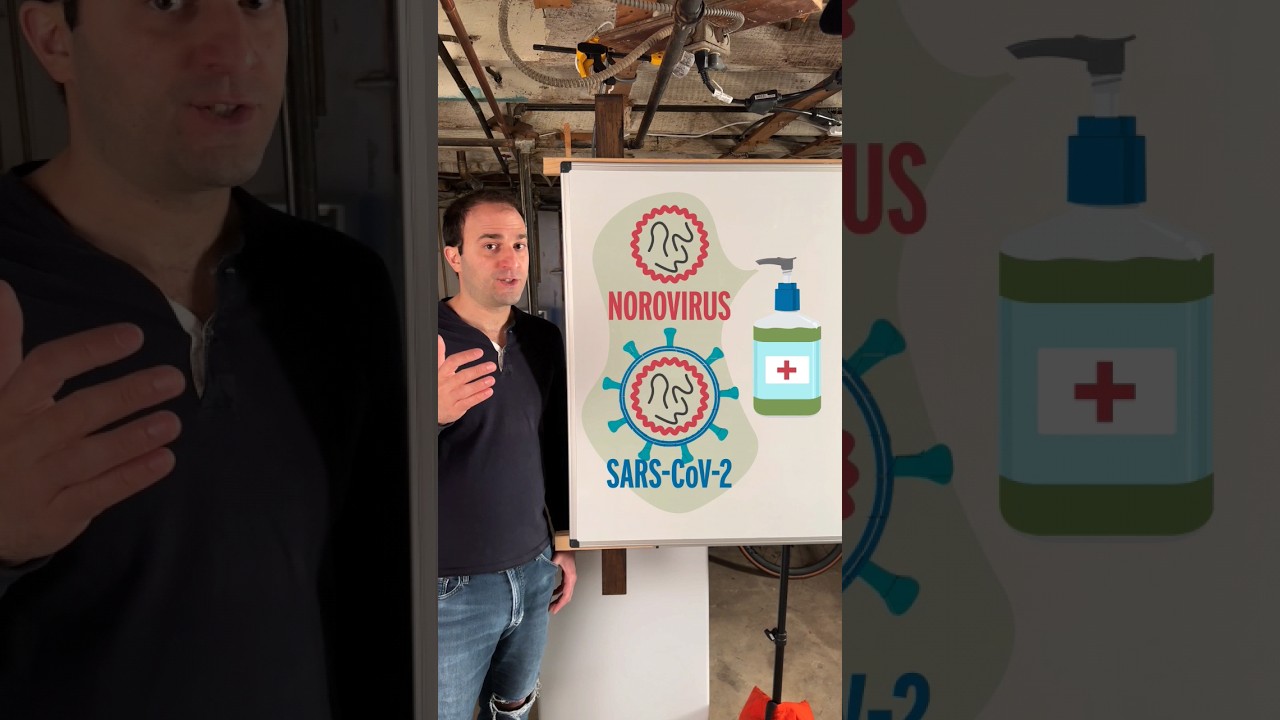
Image taken from the YouTube channel Reactions , from the video titled Enveloped vs non-enveloped viruses .
Unmasking Enveloped Viruses: A Hidden Threat
Viruses, the quintessential microscopic invaders, occupy a unique space in the biological world. They are neither truly alive nor definitively dead, existing as complex assemblies of genetic material encased in a protective protein shell, called a capsid. These entities, ranging in size from a mere 20 nanometers to several hundred, are obligate intracellular parasites. This means they can only replicate within the living cells of a host organism. Their impact on the biological world, including human health, is profound.
Within the vast virosphere exists a particularly insidious class known as enveloped viruses. Unlike their "naked" counterparts, enveloped viruses possess an additional layer of protection: a lipid bilayer membrane derived from the host cell they infected. This seemingly simple addition grants them significant advantages, turning them into a formidable and often elusive enemy.
The Enigmatic Envelope
The envelope itself is not merely a passive shield. Studded within this membrane are viral proteins, often glycoproteins, which play a crucial role in the virus's life cycle. These proteins mediate attachment to host cells, facilitate entry, and can even contribute to immune evasion. Understanding the structure and function of the viral envelope is therefore paramount to understanding the threat posed by these viruses.
A Hidden Threat Revealed
Enveloped viruses are considered a "hidden threat" for several reasons. Their reliance on host cell membranes for their envelopes allows them to exhibit a degree of molecular mimicry. This can complicate the immune system's ability to recognize and neutralize the virus. The envelope itself can also be a target for antibodies, but its fluid nature and the ability of the virus to shed its surface proteins provide a mechanism for escape.
Furthermore, the very process of budding, by which enveloped viruses exit the host cell, can be relatively non-lytic. The infected cell can remain alive and continue producing viral particles for an extended period. This prolonged, often subtle infection can delay diagnosis and contribute to the spread of the virus.
Enveloped viruses include some of the most devastating pathogens known to humankind. These pathogens cause diseases ranging from the common cold and influenza to life-threatening conditions like AIDS, Ebola hemorrhagic fever, and COVID-19. The ability of these viruses to mutate rapidly, coupled with their mechanisms for immune evasion, makes them a constant challenge for public health and biomedical research.
Understanding the unique characteristics of enveloped viruses, from their structure and life cycle to their interactions with the host immune system, is essential for developing effective strategies to combat these persistent and evolving threats.
The capacity of enveloped viruses to mimic host cells presents significant challenges to the immune system, making them adept at evading detection and establishing infection. To fully appreciate the nature of this "hidden threat," a deeper understanding of their structure and origins is essential.
Defining Enveloped Viruses: Structure and Origin
Enveloped viruses are defined by the presence of a lipid bilayer membrane surrounding their capsid, the protein shell that encases their genetic material. This envelope is not encoded by the virus itself, but is instead derived from the host cell membrane during the viral budding process. This unique characteristic distinguishes them from non-enveloped, or "naked," viruses.
The Lipid Bilayer: A Stolen Cloak
The lipid bilayer that forms the viral envelope is essentially a fragment of the host cell's own membrane. As the virus buds out of the host cell, it wraps itself in a portion of the cell's outer membrane, effectively stealing a cloak of invisibility.
The composition of the lipid bilayer is largely determined by the type of host cell the virus infects. This can lead to variations in the envelope's lipid content and fluidity. The envelope isn't a perfect copy. The virus carefully selects specific lipids and proteins to incorporate, making the envelope a tailored interface with the outside world.
This acquisition of host cell membrane is a crucial step in the viral life cycle. It allows the virus to avoid immediate recognition by the host's immune system.
Viral Envelope Proteins: Keys to Entry and Immune Evasion
Embedded within the lipid bilayer are viral envelope proteins. These proteins are encoded by the viral genome and are critical for the virus's ability to infect new cells. They perform a variety of functions, including:
-
Attachment to host cells: Envelope proteins mediate the initial binding of the virus to specific receptors on the surface of the host cell. This interaction determines the virus's host range and tissue tropism.
-
Facilitating cell entry: After attachment, envelope proteins trigger the fusion of the viral envelope with the host cell membrane, allowing the virus to enter the cell. Some viruses enter via endocytosis.
-
Immune evasion: Some envelope proteins can interfere with the host's immune response, further enhancing the virus's ability to establish infection. These proteins can mask viral antigens. Others can actively suppress immune cell function.
Spike Proteins and Hemagglutinin: Prominent Examples
Two well-known examples of viral envelope proteins are Spike proteins, found on coronaviruses like SARS-CoV-2, and Hemagglutinin, found on influenza viruses.
Spike proteins mediate the entry of SARS-CoV-2 into host cells by binding to the ACE2 receptor. Similarly, Hemagglutinin facilitates the attachment of influenza viruses to host cells and promotes viral entry.
Both Spike proteins and Hemagglutinin are major targets for neutralizing antibodies. This is why they are key components of many vaccines. Their critical role also makes them prime targets for antiviral drug development.
Understanding the structure and function of these viral envelope proteins is crucial for developing effective strategies to combat enveloped virus infections. Their interactions with the host cell and the immune system are key to understanding the virus's success.
The envelope, artfully stolen from the host, becomes a dynamic interface, mediating the virus's interaction with the next target. But how does this interaction unfold? How does the virus, cloaked in borrowed membrane, actually initiate infection and propagate? The answer lies in the intricate, step-by-step process that defines the enveloped virus life cycle.
The Enveloped Virus Life Cycle: A Step-by-Step Breakdown
The life cycle of an enveloped virus is a precisely orchestrated sequence of events, each step crucial for successful infection and propagation. From the initial attachment to a host cell to the final release of newly assembled virions, the virus navigates a complex landscape of cellular machinery and immune defenses.
Receptor Binding: The Key to Host Cell Specificity
The first critical step in the viral life cycle is receptor binding.
This is where the virus attaches to the host cell.
This attachment isn't random; it's a highly specific interaction between viral surface proteins and receptor molecules on the host cell membrane.
Think of it as a lock-and-key mechanism: the viral protein (the key) must perfectly match the host cell receptor (the lock) for binding to occur.
This specificity determines which types of cells a virus can infect, dictating its host range and tissue tropism.
For example, influenza viruses bind to sialic acid receptors, which are found on the surface of respiratory epithelial cells, explaining why these viruses primarily infect the respiratory tract.
The affinity and avidity of this interaction are vital factors that determine the efficiency of infection.
Cell Entry: Gaining Access to the Cellular Interior
Once the virus has attached to its target cell, it must enter the cell to initiate replication. Enveloped viruses employ two primary mechanisms to achieve this: membrane fusion and endocytosis.
Membrane Fusion: A Direct Route
In membrane fusion, the viral envelope directly fuses with the host cell membrane, releasing the viral capsid into the cytoplasm.
This process is typically triggered by a conformational change in viral fusion proteins, which are activated by receptor binding or changes in pH.
Fusion allows the virus to bypass the endosomal pathway, delivering its genetic material directly into the cellular environment.
Endocytosis: An Indirect Route
Endocytosis involves the virus being engulfed by the host cell membrane, forming a vesicle called an endosome.
The virus is then internalized within this vesicle.
To escape the endosome, the virus must disrupt the vesicle membrane, often through pH-dependent mechanisms or specialized viral proteins.
This releases the viral capsid into the cytoplasm.
The choice of entry mechanism can vary depending on the virus and the type of host cell.
Viral Replication: Hijacking Cellular Machinery
Once inside the host cell, the virus commandeers the cellular machinery to replicate its genetic material and produce viral proteins. The specifics of this process depend on the type of viral genome (DNA or RNA) and its replication strategy.
-
DNA viruses often utilize the host cell's DNA polymerase to replicate their genome in the nucleus.
-
RNA viruses, on the other hand, typically encode their own RNA-dependent RNA polymerase to replicate their genome in the cytoplasm.
This replication process can be highly error-prone, leading to the generation of mutations and the emergence of new viral variants.
The newly synthesized viral proteins are then translated by the host cell's ribosomes, further depleting the cell's resources.
Assembly and Budding: Acquiring the Envelope
The final stages of the viral life cycle involve the assembly of new viral particles and their release from the host cell.
Viral proteins and replicated genomes migrate to the assembly sites within the cell.
There, they self-assemble into new viral capsids.
In the case of enveloped viruses, the capsid then buds through the host cell membrane, acquiring its envelope in the process.
During budding, specific viral envelope proteins are incorporated into the host cell membrane, forming the characteristic spikes or projections on the viral surface.
This process can be highly disruptive to the host cell membrane, often leading to cell damage or death.
The newly formed virions are then released from the host cell, ready to infect new cells and continue the cycle of infection.
This budding process is also how the virus 'steals' the host cell's membrane, as the virus buds out, the host cell membrane surrounds the virus, thus completing the replication cycle.
The viral life cycle, with its elegant choreography of attachment, entry, replication, and release, paints a picture of relentless biological imperative. But the true significance of these processes becomes starkly clear when we consider specific examples of enveloped viruses and the profound impact they have on global health.
Enveloped Virus Spotlight: Notable Examples and Their Impact
Examining specific enveloped viruses offers insights into their unique characteristics, the diseases they cause, and their far-reaching global consequences. Each virus presents a unique challenge, shaped by its structure, replication strategy, and interaction with the host immune system.
Coronaviruses: SARS-CoV-2 and COVID-19
Coronaviruses are a large family of RNA viruses, some of which cause disease in humans. Among them, SARS-CoV-2, the virus responsible for the COVID-19 pandemic, has had an unprecedented global impact.
The virus is characterized by its distinctive spike proteins, which mediate entry into host cells by binding to the ACE2 receptor.
The rapid spread of SARS-CoV-2, coupled with its varying degrees of severity, placed immense strain on healthcare systems worldwide.
Beyond the immediate health crisis, the pandemic has also triggered significant economic and social disruption, highlighting the far-reaching consequences of viral emergence.
Influenza Viruses: A Seasonal Threat
Influenza viruses, another group of RNA viruses, are responsible for seasonal epidemics of the flu.
These viruses are characterized by their surface glycoproteins, hemagglutinin (HA) and neuraminidase (NA), which are essential for viral entry and release, respectively.
Antigenic drift, the gradual accumulation of mutations in HA and NA, allows influenza viruses to evade the immune system, necessitating annual vaccine updates.
Antigenic shift, a more dramatic genetic reassortment, can lead to the emergence of novel influenza strains with pandemic potential, as seen with the 1918 Spanish flu and the 2009 H1N1 pandemic.
The constant evolution of influenza viruses underscores the need for ongoing surveillance and vaccine development efforts.
HIV: The AIDS Pandemic
Human immunodeficiency virus (HIV) is a retrovirus that causes acquired immunodeficiency syndrome (AIDS). As a retrovirus, HIV uses reverse transcriptase to convert its RNA genome into DNA, which is then integrated into the host cell's genome.
HIV primarily infects CD4+ T cells, which are critical components of the immune system.
The gradual depletion of CD4+ T cells leads to immune dysfunction, making individuals susceptible to opportunistic infections and certain cancers.
While there is no cure for HIV, antiretroviral therapy (ART) can effectively suppress viral replication, allowing individuals with HIV to live long and healthy lives.
However, access to ART remains a challenge in many parts of the world, highlighting the ongoing global health disparities associated with HIV/AIDS.
Herpesviruses: A Persistent Presence
Herpesviruses are a large family of DNA viruses that are known for their ability to establish lifelong latent infections.
Examples include herpes simplex virus (HSV), varicella-zoster virus (VZV), and Epstein-Barr virus (EBV).
HSV causes oral and genital herpes, while VZV causes chickenpox and shingles.
EBV is associated with infectious mononucleosis and certain types of cancer.
Herpesviruses can reactivate from latency under conditions of stress or immune suppression, leading to recurrent disease.
The persistence of herpesviruses within the host highlights the complex interplay between viruses and the immune system.
Hepatitis B Virus: A Chronic Liver Infection
Hepatitis B virus (HBV) is a DNA virus that causes liver infection.
Chronic HBV infection can lead to cirrhosis, liver failure, and hepatocellular carcinoma.
HBV is transmitted through blood and bodily fluids, and vaccination is an effective means of prevention.
Antiviral drugs can help to suppress HBV replication and prevent liver damage, but a cure remains elusive for chronic HBV infection.
Ebola Virus: A Hemorrhagic Fever
Ebola virus is an RNA virus that causes severe hemorrhagic fever.
Ebola virus is transmitted through direct contact with infected bodily fluids.
Ebola outbreaks are characterized by high mortality rates and can cause significant disruption to healthcare systems.
The 2014-2016 West Africa Ebola epidemic highlighted the challenges of controlling viral outbreaks in resource-limited settings.
Zika Virus: Congenital Disabilities
Zika virus is an RNA virus that is primarily transmitted by mosquitoes.
Zika virus infection during pregnancy can cause severe congenital disabilities, including microcephaly.
Zika outbreaks have raised concerns about the potential for mosquito-borne viruses to cause significant public health problems.
Other Notable Enveloped Viruses
In addition to the viruses discussed above, other notable enveloped viruses include:
- Measles virus (RNA)
- Mumps virus (RNA)
- Rubella virus (RNA)
- Rabies virus (RNA)
These viruses highlight the diversity of enveloped viruses and their impact on human health.
Understanding the characteristics, transmission routes, and disease mechanisms of these viruses is crucial for developing effective prevention and treatment strategies.
The viral life cycle, with its elegant choreography of attachment, entry, replication, and release, paints a picture of relentless biological imperative. But the true significance of these processes becomes starkly clear when we consider specific examples of enveloped viruses and the profound impact they have on global health.
The Immune System vs. Enveloped Viruses: A Battle for Supremacy
The human body is not defenseless against the onslaught of enveloped viruses. A complex and sophisticated immune system stands ready to detect, neutralize, and eliminate these invaders.
This intricate battle between virus and host determines the severity and duration of infection, highlighting the critical importance of understanding the immune response to enveloped viruses.
The Immune Response: A Multi-pronged Attack
The immune system mounts a multifaceted response to combat enveloped viruses, utilizing both innate and adaptive immunity. The innate immune system provides the first line of defense, with cells like macrophages and natural killer (NK) cells recognizing viral components and initiating an inflammatory response.
This initial response aims to contain the infection and alert the adaptive immune system. The adaptive immune system, characterized by its ability to learn and remember specific pathogens, then takes center stage.
Antibodies: Precision-Guided Missiles
Antibodies, produced by B-cells, are crucial for neutralizing enveloped viruses. These Y-shaped proteins bind specifically to viral surface proteins, such as the spike protein of SARS-CoV-2 or the hemagglutinin (HA) of influenza viruses.
This binding can prevent the virus from attaching to and entering host cells, effectively neutralizing its infectivity. Antibodies can also mark infected cells for destruction by other immune cells, such as macrophages, through a process called antibody-dependent cellular cytotoxicity (ADCC).
The production of high-affinity, neutralizing antibodies is a key goal of vaccination strategies.
T-Cells: Cellular Assassins
T-cells play a critical role in clearing viral infections, particularly those that have already infected cells. Cytotoxic T lymphocytes (CTLs), also known as killer T-cells, recognize viral antigens presented on the surface of infected cells and directly kill those cells.
This process eliminates the viral reservoir and prevents further viral replication. Helper T-cells, on the other hand, support the immune response by releasing cytokines that activate other immune cells, including B-cells and CTLs.
The coordinated action of CTLs and helper T-cells is essential for a robust and effective antiviral immune response.
Vaccines: Training the Immune System
Vaccines represent a powerful tool in the fight against enveloped viruses. By exposing the immune system to viral antigens in a safe and controlled manner, vaccines prime the immune system to respond quickly and effectively upon subsequent exposure to the actual virus.
Vaccines can elicit both antibody and T-cell responses, providing long-lasting protection against infection or reducing the severity of disease. Different vaccine technologies, such as mRNA vaccines and viral vector vaccines, have shown remarkable success in combating enveloped viruses like SARS-CoV-2.
The development and deployment of effective vaccines are crucial for controlling viral epidemics and pandemics.
Antiviral Drugs: Directing Attacking the Virus
Antiviral drugs offer another line of defense against enveloped viruses. These medications work by directly targeting viral processes, such as replication or entry into host cells.
For example, some antiviral drugs inhibit viral enzymes, like the reverse transcriptase of HIV, while others block viral entry by interfering with the binding of viral proteins to host cell receptors.
Antiviral drugs can help to reduce viral load, alleviate symptoms, and prevent complications of viral infections. However, the development of antiviral resistance remains a significant challenge.
The development and deployment of vaccines and antiviral medications represent powerful tools in our arsenal against enveloped viruses. However, the story doesn’t end there. We must also consider one of the most formidable challenges in virology: viral mutation.
Viral Mutation: The Ever-Evolving Threat
Viruses, particularly RNA viruses, are masters of adaptation. Their genetic material is prone to errors during replication, leading to a constant stream of mutations. This inherent mutability poses a significant challenge to both natural immunity and medical interventions. It represents a continuously shifting landscape where yesterday’s solutions may be rendered ineffective tomorrow.
The Mechanics of Viral Mutation
Viral mutation occurs when there are changes in the genetic material (RNA or DNA) of a virus. These changes can arise due to several factors. These include errors during replication, recombination, or the effects of mutagens. RNA viruses, such as influenza and coronaviruses, tend to have higher mutation rates. This is because their RNA polymerases lack the proofreading capabilities of DNA polymerases.
These mutations can be single nucleotide changes, insertions, or deletions. The accumulation of these changes over time can lead to significant alterations in the virus’s characteristics. These alterations include its ability to infect cells, cause disease, and evade the immune system.
The Emergence of New Viral Strains
The constant accumulation of mutations can lead to the emergence of new viral strains. A strain is a genetic variant of a virus that has distinct characteristics compared to the original virus. These characteristics may include increased transmissibility, virulence, or resistance to antiviral drugs.
Antigenic drift is a gradual process of mutation that occurs over time. This results in small changes in the virus's surface proteins. Antigenic shift, on the other hand, is a more abrupt and significant change. This often occurs through genetic reassortment. This is where two or more different viral strains combine their genetic material to form a new subtype. Both of these processes can result in the emergence of new viral strains that are different enough from previous strains.
Impact on the Immune Response
Viral mutations can significantly impact the effectiveness of the immune response. Antibodies, generated in response to a specific viral strain, bind to viral surface proteins and neutralize the virus. If mutations alter these surface proteins, the antibodies may no longer be able to bind effectively. This phenomenon is known as immune escape.
T-cells, another critical component of the adaptive immune system, can also be affected by viral mutations. If viral mutations alter the viral peptides presented by infected cells, T-cells may no longer recognize and kill the infected cells. This can lead to prolonged infections and increased disease severity.
Challenges to Vaccine Effectiveness
Vaccines are designed to elicit an immune response that protects against specific viral strains. However, the emergence of new viral strains through mutation can reduce the effectiveness of vaccines. If the mutations are significant enough, the antibodies generated by the vaccine may not be able to neutralize the new viral strain.
This is why seasonal influenza vaccines must be updated annually to match the circulating strains. Researchers must constantly monitor the evolution of influenza viruses. They then must predict which strains are most likely to circulate in the upcoming season. This allows them to update the vaccine accordingly.
The Quest for Broad-Spectrum Antivirals
Given the challenges posed by viral mutation, there is a growing need for broad-spectrum antivirals. These antivirals would target viral mechanisms that are conserved across different viral strains. These conserved mechanisms are less likely to be affected by mutation.
Developing such antivirals is a significant challenge. Viruses are highly diverse. Finding a target that is both essential for viral replication and conserved across different viruses is difficult. However, ongoing research efforts are focused on identifying and developing these broad-spectrum antivirals.
Future Directions
The ongoing battle against enveloped viruses requires a multi-pronged approach. This approach must include the development of new vaccines and antiviral drugs. It must also include improved surveillance and monitoring of viral evolution.
Understanding the mechanisms of viral mutation is crucial for predicting the emergence of new viral strains. It is also crucial for designing effective strategies to combat them. By staying one step ahead of viral evolution, we can better protect ourselves against the ever-evolving threat of enveloped viruses.
The emergence of new viral strains highlights the virus's relentless ability to adapt and overcome obstacles. Understanding these genetic shifts is paramount, but so is understanding how these viruses spread and how we can protect ourselves.
Viral Transmission and Prevention Strategies
Enveloped viruses employ diverse transmission strategies, each presenting unique challenges for prevention. Understanding these routes is crucial for implementing effective control measures and mitigating the risk of infection.
Modes of Viral Transmission
Enveloped viruses exhibit a range of transmission pathways, broadly categorized into:
-
Respiratory Droplets: Many enveloped viruses, including influenza and coronaviruses, spread through respiratory droplets expelled during coughing, sneezing, talking, or even breathing. These droplets can travel short distances and infect individuals who inhale them directly or touch contaminated surfaces.
-
Bodily Fluids: Viruses like HIV, Hepatitis B, and Ebola can transmit through direct contact with infected bodily fluids, such as blood, semen, vaginal fluids, and breast milk. This mode of transmission emphasizes the importance of safe practices in healthcare settings, as well as responsible sexual behavior and avoidance of intravenous drug use with shared needles.
-
Vertical Transmission: Certain enveloped viruses, like Zika virus, can transmit from a pregnant mother to her fetus during pregnancy or delivery. This vertical transmission can result in severe congenital disabilities, highlighting the need for preventative measures in pregnant women residing in or traveling to areas with known viral outbreaks.
-
Vector-borne Transmission: Arboviruses, such as Zika virus and West Nile virus, are transmitted through the bites of infected arthropod vectors, like mosquitoes and ticks. Control measures targeting the vector population are essential to prevent the spread of these viruses.
-
Direct Contact: Some enveloped viruses, such as herpes simplex virus (HSV), spread through direct contact with infected skin or mucous membranes. This mode of transmission underscores the importance of practicing good hygiene, especially avoiding contact with visible sores or lesions.
Prevention Strategies: A Multi-Pronged Approach
Effective prevention of enveloped virus infections requires a combination of individual responsibility and public health interventions.
Hygiene Practices
Handwashing remains one of the most effective and accessible methods for preventing the spread of many enveloped viruses. Regular and thorough handwashing with soap and water can remove viral particles from the skin and prevent transmission.
Respiratory etiquette, such as covering coughs and sneezes with a tissue or elbow, also helps to minimize the spread of respiratory droplets.
Social Distancing
During outbreaks of respiratory viruses, social distancing measures can significantly reduce transmission rates. This may include avoiding crowded places, maintaining physical distance from others, and working from home when possible.
Vaccination
Vaccines are a cornerstone of prevention for many enveloped viral infections. Vaccines work by exposing the immune system to a weakened or inactivated form of the virus. This prompts the body to produce antibodies and cellular immunity, providing protection against future infection.
Vaccines are available for several enveloped viruses, including influenza, measles, mumps, rubella, varicella-zoster virus (chickenpox), and hepatitis B. Development efforts continue for vaccines against other viruses, such as HIV.
Antiviral Medications
Antiviral medications can also play a role in preventing or mitigating the severity of certain enveloped viral infections. Some antiviral drugs can prevent viral entry into cells, while others can inhibit viral replication.
These drugs can be used prophylactically to prevent infection in individuals at high risk of exposure or therapeutically to treat active infections.
Public Health Initiatives
Effective prevention also requires robust public health initiatives, including:
-
Surveillance: Monitoring the spread of viruses and identifying outbreaks early is crucial for implementing timely control measures.
-
Education: Public education campaigns can raise awareness about viral transmission and prevention strategies, empowering individuals to protect themselves and their communities.
-
Infrastructure: Investing in healthcare infrastructure, including diagnostic testing and treatment facilities, is essential for managing viral infections effectively.
By understanding the modes of transmission and implementing comprehensive prevention strategies, we can mitigate the impact of enveloped viruses and protect public health.
The ability of viruses to mutate and spread through various means necessitates a proactive rather than reactive approach. Understanding the nuances of viral transmission allows us to implement targeted preventative measures, mitigating the risk of infection.
Future Horizons: Ongoing Research and Novel Therapies
The fight against enveloped viruses is far from over. Scientific advancements are constantly reshaping our understanding and treatment of these pathogens. The future hinges on sustained research, innovative therapies, and proactive global surveillance to stay ahead of emerging threats.
Persistent Research Efforts
Ongoing research encompasses a wide array of investigations aimed at unraveling the complexities of enveloped viruses. This includes studies focused on:
-
Viral Structure and Function: Detailed analysis of viral proteins and their interactions with host cells.
- This deeper understanding helps identify potential targets for drug development and vaccine design.
-
Immune Responses: Investigating how the human immune system responds to enveloped viruses.
- Research aims to identify the key components of effective immunity and develop strategies to enhance these responses.
-
Viral Evolution: Monitoring viral mutations and tracking the emergence of new variants.
- Understanding how viruses evolve is essential for predicting future threats and adapting existing countermeasures.
Novel Vaccine and Antiviral Development
The development of new vaccines and antiviral therapies remains a central focus in combating enveloped viruses. Current research is exploring:
-
Next-Generation Vaccines: Moving beyond traditional vaccine approaches to develop more effective and broadly protective vaccines.
- This includes mRNA vaccines, viral vector vaccines, and subunit vaccines.
- These next-generation approaches often elicit stronger and more durable immune responses.
-
Broad-Spectrum Antivirals: Developing drugs that can target multiple viruses or viral strains.
- This is crucial for addressing the challenge of viral mutation.
- These antivirals could potentially be used to treat infections caused by newly emerging viruses.
-
Immunotherapies: Harnessing the power of the immune system to fight viral infections.
-
This includes strategies such as monoclonal antibodies and adoptive T-cell therapy.
-
These novel approaches offer the potential to provide targeted and effective antiviral immunity.
-
Global Surveillance and Preparedness
Global surveillance is essential for detecting emerging viral threats early and responding quickly to outbreaks. This involves:
- Monitoring Viral Activity: Tracking the spread of viruses in human and animal populations.
-
Rapid Diagnostics: Developing and deploying rapid diagnostic tests to identify viral infections quickly and accurately.
- This allows for prompt implementation of control measures.
-
International Collaboration: Fostering collaboration between researchers, public health organizations, and governments around the world.
- Sharing information and resources is crucial for effective global surveillance and preparedness.
By investing in research, developing innovative therapies, and strengthening global surveillance efforts, we can better prepare for future viral threats and protect public health. The ongoing pursuit of knowledge is our strongest weapon in this ongoing battle.
Video: Enveloped Virus: Unveiling the Hidden Threat (You Must Know)
FAQs: Understanding Enveloped Viruses
Here are some frequently asked questions to help you better understand enveloped viruses and the threats they pose.
What exactly makes a virus an "enveloped" virus?
An enveloped virus is one that has a protective outer layer, called an envelope. This envelope is derived from the host cell's membrane during the virus's exit. It helps the enveloped virus evade the host's immune system and infect other cells.
How does the envelope help an enveloped virus spread?
The envelope contains proteins that allow the enveloped virus to bind to and enter new host cells. It also makes the virus more fragile outside the host, as the envelope can be easily damaged by detergents and disinfectants.
Are enveloped viruses more dangerous than non-enveloped viruses?
Not necessarily. While the envelope aids in entry, it also makes the enveloped virus more susceptible to certain disinfectants and environmental conditions. The danger of a virus depends on many factors, including its infectivity, pathogenicity, and the availability of treatments or vaccines.
What are some common examples of enveloped viruses?
Many well-known viruses are enveloped. Examples include influenza viruses (the flu), HIV (which causes AIDS), herpes viruses, and coronaviruses like SARS-CoV-2 (which causes COVID-19).