Electron Charge: Unveiling Its Secrets (Must Read!)
The fundamental property, electron charge, dictates much of the universe's behavior, from the interactions within a single atom to the vast phenomena studied at CERN's Large Hadron Collider. Understanding electron charge is key to mastering concepts in quantum mechanics and its implications in the development of modern semiconductors. Experiments pioneered by Robert Millikan provided foundational measurements of the electron charge, solidifying its place in the Standard Model of particle physics. Indeed, exploring the secrets of electron charge unlocks a deeper understanding of how electricity works.
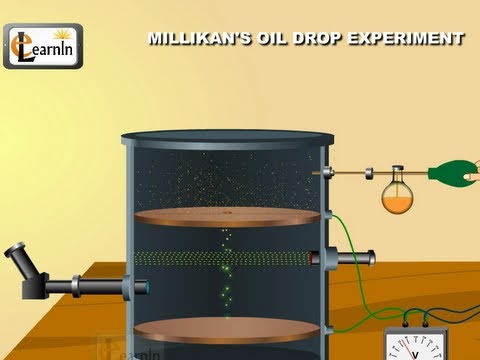
Image taken from the YouTube channel Elearnin , from the video titled Millikan's oil drop experiment to determine charge of an electron - Chemistry .
The universe, in its breathtaking complexity, often yields its deepest secrets to those who diligently explore its smallest components. Among these, the electron, with its inherent electric charge, stands as a cornerstone of reality. Understanding this fundamental property isn't merely an academic pursuit; it's a key to unlocking the intricate workings of everything from the smallest atoms to the grandest cosmic structures.
Why Electron Charge Matters
Imagine trying to build a house without understanding the properties of bricks or cement. Similarly, without grasping electron charge, we remain fundamentally limited in our understanding of matter and energy.
Electron charge dictates how atoms interact, forming molecules, and ultimately, everything we see and touch. It's the invisible hand shaping chemical reactions, the engine driving electrical currents, and the architect behind the very fabric of our physical world.
Defining Electric Charge: The Foundation of Electromagnetism
Electric charge, at its core, is a fundamental property of matter that causes it to experience a force when placed in an electromagnetic field. It's the characteristic that dictates how a particle interacts with photons, the force carriers of electromagnetism.
This interaction is responsible for nearly all the phenomena we experience daily, from the light that allows us to see to the technologies that power our modern lives. Understanding electric charge is therefore inseparable from understanding electromagnetism, one of the four fundamental forces of nature.
Furthermore, electric charge is not just about force; it’s about structure. The balance between positively charged protons in the nucleus of an atom and negatively charged electrons orbiting that nucleus dictates the atom's stability and its ability to form bonds with other atoms. This balance is crucial.
Thesis: A Journey into the Heart of Matter
This article embarks on a comprehensive journey to explore the multifaceted nature of electron charge. From its foundational definition and historical measurement to its profound significance in modern physics and technological applications, we aim to provide a holistic understanding of this essential property.
We will delve into the groundbreaking experiments that revealed its value, its pivotal role in atomic structure and chemical bonding, and its modern interpretation within the framework of quantum mechanics. Ultimately, we seek to illuminate why understanding electron charge remains not just relevant, but absolutely essential for continued scientific progress and technological innovation.
The balance between positively charged protons in the nucleus of an atom and negatively charged electrons buzzing around it is a delicate dance that dictates the very essence of matter. Without understanding the electron’s role in this intricate choreography, we're left with an incomplete picture of the universe’s fundamental building blocks. So, let's delve deeper into what exactly electron charge is and why it's so important.
What is Electron Charge? The Building Block of Matter
At the heart of understanding matter lies the concept of electron charge.
It’s a fundamental property, an intrinsic characteristic that defines how electrons interact with the electromagnetic force. It’s the very essence of what makes an electron an electron.
The Electron: An Indivisible Particle
The electron is a fundamental particle, meaning it isn't composed of smaller, constituent particles. It's one of the indivisible components of atoms, the basic units of matter.
Think of it as a single, irreducible unit, a point-like entity carrying a specific amount of negative charge. Unlike composite particles like protons or neutrons, which are made up of quarks, the electron stands alone.
Its existence is a given, a foundational element upon which the entire structure of matter is built.
Elementary Charge: The Quantum of Electricity
The concept of elementary charge is crucial. It represents the smallest unit of electric charge that can exist freely.
It's the magnitude of the charge carried by a single proton or electron.
While protons carry a positive elementary charge and electrons carry a negative elementary charge, the magnitude of both is identical. Denoted by the symbol e, its value is approximately 1.602 × 10⁻¹⁹ Coulombs.
This constant, e, is a cornerstone of physics, appearing in countless equations and calculations across electromagnetism and quantum mechanics.
Charge Quantization: Discrete Packets of Electricity
Electric charge isn't continuous; it's quantized. This means that charge doesn't come in any arbitrary amount.
Instead, it exists in discrete multiples of the elementary charge, e.
You can have a charge of e, 2e, 3e, and so on, but never 1.5e or any other fractional amount. This is a profound observation with significant implications.
Think of it like money. You can have one dollar, two dollars, or three dollars, but you can't have 2.5 dollars in cash (though you can in a bank account, which is a different analogy altogether!). Electric charge is similar; it comes in specific, defined packets.
This quantization of charge is not just a curiosity, but a fundamental law of nature, deeply ingrained in the fabric of reality. It governs how particles interact and how matter is structured at its most basic level.
The electron's indivisible nature and its possession of the elementary charge beg the question: how do we even begin to quantify something so infinitesimally small? How did scientists transition from theoretical postulations to concrete measurements of this fundamental constant? The answer lies in a beautifully ingenious experiment that bridged the gap between the abstract world of particle physics and tangible observation.
Measuring the Infinitesimal: Millikan's Oil Drop Experiment
Robert Andrews Millikan, an American physicist, stands as a pivotal figure in the history of science due to his meticulous determination of the elementary electric charge. His Oil Drop Experiment, conducted between 1909 and 1913, wasn't merely a laboratory procedure; it was a watershed moment that solidified our understanding of the quantized nature of electricity.
The Significance of Millikan's Work
Before Millikan's experiment, the existence of electrons was known, and their negative charge understood qualitatively. However, the precise value of that charge remained elusive. Millikan's work provided the first accurate and direct measurement, earning him the Nobel Prize in Physics in 1923.
His experiment demonstrated definitively that electric charge is not continuous but exists in discrete units – multiples of the elementary charge. This was a crucial confirmation of the atomic theory of matter and paved the way for further advancements in quantum physics.
Deconstructing the Oil Drop Experiment
The beauty of Millikan's experiment lies in its elegant simplicity. He used an atomizer to spray tiny oil droplets into a chamber. These droplets, due to friction, acquired a small electric charge, sometimes positive, but more often negative.
These charged droplets then fell between two horizontal metal plates, where Millikan could control the electric field. By carefully adjusting the voltage between the plates, he could counteract the force of gravity acting on the droplets.
When the electric force perfectly balanced the gravitational force, the droplet would hover suspended in mid-air. Millikan could then calculate the magnitude of the electric charge on the droplet using the known electric field strength and the droplet's mass.
Unveiling the Quantum Nature of Charge
Millikan didn't just measure the charge of a single droplet. He repeated the experiment countless times, measuring the charges on many different droplets. The crucial observation was that all the measured charges were always integer multiples of a single, smallest value.
For example, a droplet might have a charge of -1.602 x 10-19 Coulombs (the charge of one electron), another might have -3.204 x 10-19 Coulombs (the charge of two electrons), and so on.
This demonstrated that electric charge is quantized. It comes in discrete packets, the smallest of which is the elementary charge, e.
The Coulomb: Quantifying Electric Charge
The standard unit of electric charge in the International System of Units (SI) is the Coulomb, named after French physicist Charles-Augustin de Coulomb. One Coulomb is defined as the amount of charge transported by a current of one ampere in one second.
Millikan's experiment allowed scientists to relate the elementary charge, e, to the Coulomb. The currently accepted value of the elementary charge is approximately 1.602176634 × 10-19 Coulombs. This means that one Coulomb is equivalent to approximately 6.241509074 × 1018 elementary charges.
Millikan's experiment not only provided a precise value for the elementary charge but also cemented the concept of charge quantization, a cornerstone of modern physics. His work continues to be celebrated as a triumph of experimental ingenuity and a crucial step in unraveling the mysteries of the universe at its most fundamental level.
Millikan’s meticulous experiment delivered a precise measurement of the fundamental unit of charge. But the story doesn't end with quantifying this infinitesimal value. The real magic unfolds when we examine how this fundamental charge orchestrates the very structure of matter, dictating how atoms form, interact, and ultimately, create the complexity we observe around us.
Electron Charge and the Atomic Structure
Electron charge is not merely a static property; it's the dynamic force that sculpts the atomic and molecular world. Its influence extends from the stability of individual atoms to the intricate dance of chemical bonding.
The Electron's Role in Atomic Formation and Stability
Atoms, the building blocks of matter, owe their existence and stability to the presence of electrons and their inherent negative charge. Without the electron, the positively charged nucleus would be an isolated entity.
The negative charge of electrons creates an electrostatic attraction towards the positive charge of the protons in the nucleus. This attraction is what binds the electrons to the atom, creating a stable system.
The number of electrons dictates the chemical identity of an atom. Atoms with the same number of protons but different numbers of neutrons are isotopes, but changing the electron count transforms the element entirely.
Balancing Act: Protons, Electrons, and Electrostatic Equilibrium
The stability of an atom hinges on a delicate balance between the positively charged protons residing in the nucleus and the negatively charged electrons orbiting around it.
In a neutral atom, the number of protons and electrons is precisely equal, resulting in a net charge of zero. This equilibrium is crucial for maintaining the atom's integrity.
Any imbalance in this equilibrium results in the formation of ions – atoms with a net positive or negative charge. These ions play vital roles in chemical reactions and biological processes.
Electron Charge and Chemical Bonding: The Molecular Dance
Electron charge is the choreographer of chemical bonding, dictating how atoms interact to form molecules. The sharing or transfer of electrons between atoms leads to the formation of different types of chemical bonds.
Covalent bonds arise when atoms share electrons to achieve a stable electron configuration. This sharing creates a strong attractive force, holding the atoms together.
Ionic bonds occur when one atom transfers electrons to another, creating positively and negatively charged ions. The electrostatic attraction between these ions forms the bond.
The properties of molecules, such as their shape, polarity, and reactivity, are directly determined by the distribution of electron charge within the molecule.
Electromagnetism: The Unifying Force
Electron charge is inextricably linked to electromagnetism, one of the four fundamental forces of nature. The movement of electrons generates magnetic fields, and changing magnetic fields exert forces on electrons.
This interconnectedness is the foundation of countless technological applications, from electric motors and generators to radio waves and light.
The electromagnetic force, mediated by photons, governs the interactions between charged particles, influencing everything from the behavior of atoms to the structure of galaxies.
Understanding the interplay between electron charge and electromagnetism is essential for comprehending the fundamental laws that govern the universe.
Millikan’s meticulous experiment delivered a precise measurement of the fundamental unit of charge. But the story doesn't end with quantifying this infinitesimal value. The real magic unfolds when we examine how this fundamental charge orchestrates the very structure of matter, dictating how atoms form, interact, and ultimately, create the complexity we observe around us.
Electron Charge in Modern Physics: A Quantum Perspective
Our understanding of electron charge has undergone a profound transformation through the lens of modern physics. The classical view, while incredibly useful, only scratches the surface. To truly grasp the intricacies of this fundamental property, we must delve into the realm of quantum mechanics and explore its implications.
Quantum Mechanics and Electron Charge
Quantum mechanics revolutionized our understanding of the universe, revealing that particles at the subatomic level behave in ways that defy classical intuition. The electron, with its inherent charge, is no exception.
One of the key concepts in quantum mechanics is wave-particle duality.
This principle states that particles, including electrons, exhibit both wave-like and particle-like properties. This duality has profound implications for how we understand electron charge.
It's no longer simply a static quantity associated with a discrete particle. Instead, it becomes intertwined with the electron's wave function, a mathematical description of its probability distribution in space.
Another crucial concept is that of quantum fields. In quantum field theory, particles are viewed as excitations of underlying quantum fields.
The electron, therefore, is not a point-like object but rather a ripple in the electron field. Its charge is a property of this field, influencing its interactions with other fields and particles.
Quantum Electrodynamics (QED): Light and Matter's Dance
Quantum Electrodynamics, or QED, is the quantum field theory that describes the interaction of light and matter. It is arguably the most accurate theory in all of physics, making incredibly precise predictions that have been experimentally verified to astonishing degrees of accuracy.
At the heart of QED lies the interaction between electrons and photons, the fundamental particles of light. Electron charge plays a central role in this interaction.
The strength of the electromagnetic force, which governs how electrons and photons interact, is directly proportional to the electron charge. QED describes how electrons exchange photons, mediating the electromagnetic force between them.
These interactions are not simple exchanges, however.
QED predicts that the "bare" charge of an electron is actually infinite. But due to a process called renormalization, we observe a finite, measurable charge.
This renormalization is a testament to the power and complexity of QED.
QED's predictions have been confirmed by experiments to an extraordinary level of precision. For example, the theory accurately predicts the anomalous magnetic moment of the electron, a tiny deviation from its expected magnetic moment. This agreement provides strong evidence for the validity of QED and our understanding of electron charge.
Electron Charge and the Standard Model
The Standard Model of Particle Physics is our current best description of the fundamental building blocks of the universe and their interactions. Within this model, the electron is classified as a fundamental particle, a lepton.
Its charge is one of its intrinsic properties, along with its mass and spin.
The Standard Model incorporates the principles of quantum field theory, including QED. It describes how electrons interact with other fundamental particles, such as quarks and neutrinos, through the exchange of force-carrying particles called bosons.
The electron's charge dictates its interactions with photons (electromagnetic force), as well as the W and Z bosons (weak force).
The Standard Model has been remarkably successful in explaining a wide range of experimental observations. However, it is not a complete theory. It does not incorporate gravity, nor does it explain the origin of mass or the existence of dark matter and dark energy.
Nevertheless, the Standard Model provides a robust framework for understanding electron charge and its role in the fundamental interactions of nature.
Millikan’s meticulous experiment delivered a precise measurement of the fundamental unit of charge. But the story doesn't end with quantifying this infinitesimal value. The real magic unfolds when we examine how this fundamental charge orchestrates the very structure of matter, dictating how atoms form, interact, and ultimately, create the complexity we observe around us.
Practical Applications and Technological Impact of Electron Charge Understanding
Our deepened comprehension of electron charge isn't confined to theoretical physics or academic textbooks. Instead, it serves as the bedrock upon which entire technological landscapes are built. From the ubiquitous smartphones in our pockets to the sophisticated medical imaging devices in hospitals, our ability to manipulate and harness electron charge has revolutionized nearly every facet of modern life.
This section explores some of the ways we have put this understanding to use.
The Electron's Role in Modern Electronics
The modern world is powered by electronics, and at the heart of nearly every electronic device lies the controlled flow of electrons. The transistor, arguably the most important invention of the 20th century, is a prime example.
These tiny switches, which form the basis of integrated circuits and microprocessors, rely entirely on our ability to manipulate the movement of electrons through semiconductor materials.
By precisely controlling the flow of electrons, transistors enable us to perform complex calculations, store vast amounts of data, and transmit information across the globe at incredible speeds.
Semiconductors: The Foundation of Modern Technology
Semiconductors, materials with electrical conductivity between that of a conductor and an insulator, are another cornerstone of modern technology that hinges directly on our understanding of electron charge.
The behavior of electrons in these materials can be precisely tuned by introducing impurities, a process known as doping.
This allows us to create p-n junctions, the building blocks of diodes and transistors, enabling the controlled flow of current in electronic circuits.
Without the ability to manipulate electron charge in semiconductors, much of modern electronics would be impossible.
Applications Beyond Electronics
The applications of our understanding of electron charge extend far beyond traditional electronics.
In materials science, for instance, we can design new materials with specific electrical and optical properties by controlling the arrangement and behavior of electrons within their atomic structures.
This has led to the development of advanced materials used in everything from solar cells to high-performance batteries.
Ongoing Research and Future Directions
The study of electron charge and its applications is an active area of research, with scientists constantly pushing the boundaries of what is possible.
One promising area is spintronics, which seeks to exploit the intrinsic angular momentum of electrons, known as spin, in addition to their charge.
This could lead to the development of faster, more energy-efficient electronic devices.
Another exciting area is the development of new quantum materials with exotic electronic properties, such as superconductivity and topological insulation.
These materials could revolutionize energy storage, computing, and other fields.
As our understanding of electron charge deepens, we can expect to see even more groundbreaking technological advancements in the years to come. The future of innovation is inextricably linked to our ability to understand and manipulate this fundamental property of nature.
Video: Electron Charge: Unveiling Its Secrets (Must Read!)
Electron Charge FAQs: Unveiling Key Insights
Here are some frequently asked questions about electron charge to help solidify your understanding of this fundamental concept.
What exactly is electron charge?
Electron charge is a fundamental physical property of electrons that causes them to experience a force when placed in an electromagnetic field. It's a quantized value, meaning it only exists in discrete units. In essence, it’s the amount of negative electricity an electron carries.
Is electron charge the same as static electricity?
No, electron charge refers to the intrinsic property of a single electron. Static electricity, on the other hand, is an imbalance of electric charges on the surface of a material. While electrons contribute to static electricity, the concept of electron charge is about the charge carried by a single electron.
Why is electron charge important?
Electron charge governs how electrons interact with electromagnetic fields and with each other. It’s crucial for understanding chemical bonding, electrical conductivity, and countless other phenomena in physics and chemistry. Everything from the way a computer works to how the sun generates energy relies on the properties of electron charge.
How is electron charge measured?
The electron charge was famously measured by Robert Millikan in his oil drop experiment. It’s a very small value, approximately -1.602 x 10^-19 Coulombs. This value is a fundamental constant of nature, essential for many calculations in physics and related fields.