Convection Cells: Weather, Ocean Currents Explained
Convection cells, driven by temperature gradients, are fundamental drivers of Earth's dynamic systems, such as global weather patterns and ocean currents. The Hadley Cell, a major atmospheric circulation pattern, exemplifies how rising warm air at the equator and sinking cool air at 30 degrees latitude establish large-scale convection. Moreover, the movement of tectonic plates is partially driven by convection cells in the Earth's mantle. Oceanographers employ sophisticated models and data from buoys and satellites to study the complex interplay between convection cells and thermohaline circulation, which distributes heat around the globe.
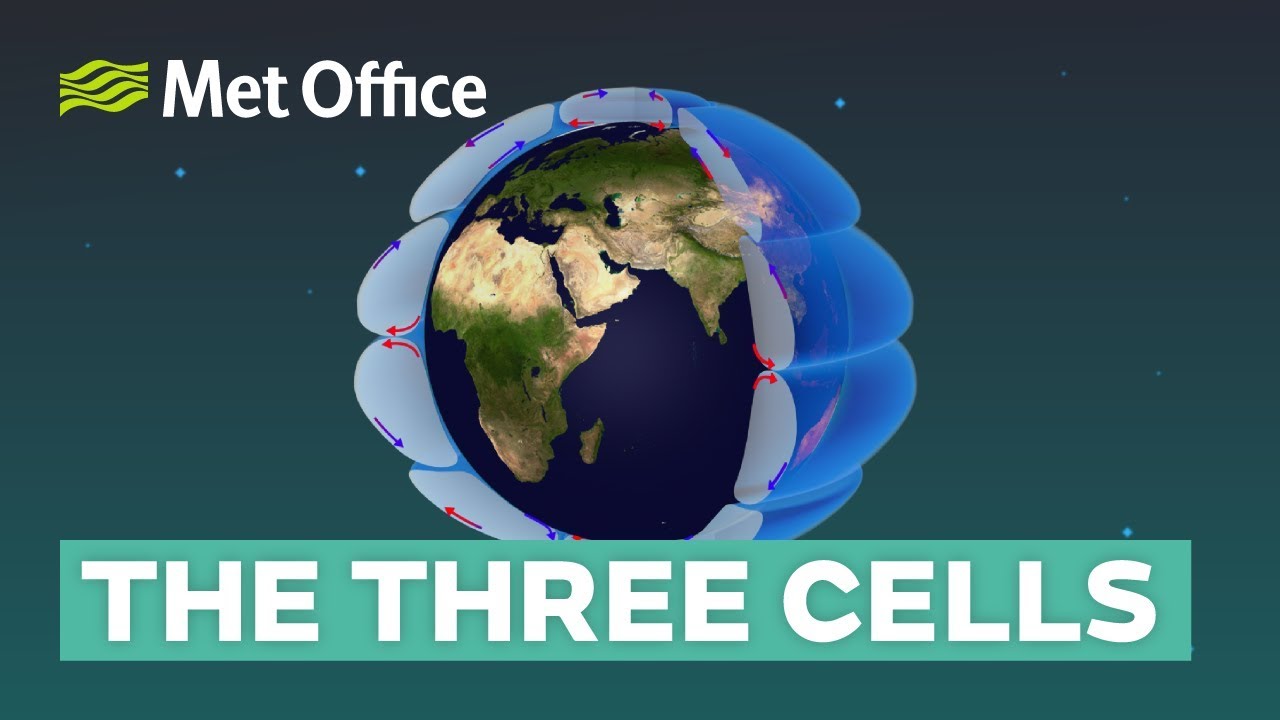
Image taken from the YouTube channel Met Office - Learn About Weather , from the video titled What is global circulation? | Part Two | The three cells .
Unveiling Convection's Ubiquitous Role on Earth
Convection, a process often unseen yet profoundly influential, stands as a cornerstone in understanding Earth's complex and dynamic systems. Its importance stems from being a primary mechanism for heat transfer. It shapes everything from atmospheric weather patterns to oceanic currents, and even the geological processes occurring deep within our planet.
Defining Convection: A Dance of Density and Heat
At its core, convection is the transfer of heat through the movement of fluids – liquids or gases. This movement arises from differences in density. These differences are, in turn, caused by variations in temperature.
When a fluid is heated, its molecules gain kinetic energy, causing them to spread out. This results in a decrease in density. The warmer, less dense fluid then rises, while the cooler, denser fluid sinks, creating a cyclical motion.
This continuous cycle of rising and sinking is what we know as a convection current. It is the fundamental principle that drives a vast array of natural phenomena.
Convection: Earth's Primary Heat Distributor
Earth's systems rely heavily on convection to redistribute thermal energy, which comes primarily from solar radiation. Without this efficient method of heat transfer, our planet would be drastically different.
Imagine a world where the equator is unbearably hot and the poles are perpetually frozen. Convection mitigates these extremes by transporting heat away from the tropics and towards higher latitudes.
This process happens in both the atmosphere and the oceans, each playing a crucial role in maintaining a global thermal equilibrium. These convection currents drive weather systems and ocean currents, and are intimately linked with maintaining a habitable environment for life as we know it.
Connecting the Dots: Convection Across Disciplines
Convection isn't confined to a single scientific discipline. Its influence extends across numerous fields, bridging the gap between seemingly disparate areas of study.
-
Meteorology: Convection is paramount in the formation of clouds, thunderstorms, and large-scale weather systems. Understanding atmospheric convection is essential for accurate weather forecasting and climate modeling.
-
Oceanography: Oceanic convection drives the global thermohaline circulation, a massive current system that distributes heat and nutrients around the world. This impacts marine ecosystems and climate regulation.
-
Geology: Deep within the Earth, mantle convection drives plate tectonics, responsible for earthquakes, volcanic activity, and the formation of mountains.
-
Environmental Science: Convection is essential in understanding and predicting pollutant dispersal in both the atmosphere and aquatic environments, making it crucial for environmental management.
The study of convection, therefore, offers a unique interdisciplinary perspective. It connects atmospheric dynamics, oceanic processes, and geological forces. It offers crucial insights into the complex workings of our planet.
The Physics of Convection: Buoyancy, Density, and Heat Transfer
[Unveiling Convection's Ubiquitous Role on Earth Convection, a process often unseen yet profoundly influential, stands as a cornerstone in understanding Earth's complex and dynamic systems. Its importance stems from being a primary mechanism for heat transfer. It shapes everything from atmospheric weather patterns to oceanic currents, and even the g...]
Understanding the intricate dance of convection demands a closer examination of its fundamental physical principles. Convection isn't merely a phenomenon; it's a consequence of well-defined physical laws governing heat transfer, buoyancy, and density. Only by dissecting these elements can we fully appreciate convection's role in Earth's diverse processes.
Dissecting Heat Transfer: Conduction, Radiation, and the Ascendancy of Convection
Heat, the engine of many natural processes, transfers energy through three primary mechanisms: conduction, radiation, and convection. Conduction involves the transfer of heat through a material via direct molecular contact. Radiation, on the other hand, transmits heat through electromagnetic waves, independent of a medium.
Convection, uniquely, relies on the movement of fluids – liquids or gases – to transfer heat. This fluid motion arises from temperature differences, leading to density variations within the fluid. While conduction and radiation play vital roles, convection stands out as the dominant mode of heat transfer in many large-scale Earth systems.
Buoyancy: The Driving Force Behind Convection
Buoyancy is the upward force exerted on an object submerged in a fluid. This force is directly linked to the density difference between the object and the surrounding fluid. In convection, warmer fluids, being less dense, experience a greater buoyant force than cooler, denser fluids.
This differential buoyancy is the engine that drives convective motion. The lighter, warmer fluid rises, displacing the heavier, cooler fluid, which in turn sinks. This continuous cycle of rising and sinking establishes a circulating flow, effectively transporting heat throughout the system.
Density Variations: The Subtle Yet Powerful Catalyst
Density, defined as mass per unit volume, is the crucial property that links temperature to convective motion. Most fluids expand when heated, causing their density to decrease. Conversely, cooling a fluid increases its density, causing it to contract.
These seemingly small density variations have profound consequences. It's the subtle interplay of temperature and density that creates the instability necessary for convective currents to form. Without density variations, there would be no buoyancy forces, and convection would cease.
Convection in Action: A Microscopic View
Imagine a pot of water being heated on a stove. The water at the bottom, closest to the heat source, warms up and becomes less dense. Buoyancy then acts upon this warmer water, causing it to rise. As the warm water ascends, cooler, denser water descends to take its place, creating a continuous cycle.
This simple example illustrates the fundamental principles of convection at work. It also highlights the complex interplay between heat transfer, buoyancy, and density that underlies this essential Earth process. Understanding these individual components is critical to explaining the global impact of convection in weather, climate, and geological processes.
Atmospheric Convection: Shaping Weather and Climate
Having established the fundamental physics of convection, we now turn our attention to its manifestation within Earth's atmosphere. Atmospheric convection stands as a pivotal force, orchestrating weather phenomena and shaping climate patterns across the globe. Its intricate interplay of heat, moisture, and air movement gives rise to the diverse atmospheric conditions we experience daily.
The Atmosphere: A Convective Engine
The atmosphere serves as a dynamic convective engine, constantly redistributing heat from the equator towards the poles.
This redistribution is not uniform; it occurs through a series of interconnected convection cells that define global weather systems.
Understanding these cells is crucial to grasping the larger picture of atmospheric circulation.
Major Atmospheric Convection Cells
The Earth’s atmosphere features three primary convection cells in each hemisphere: the Hadley Cell, the Ferrel Cell, and the Polar Cell. Each cell operates on distinct principles, contributing uniquely to global heat distribution.
The Hadley Cell: Tropical Dominance
The Hadley Cell, closest to the equator, is driven by intense solar heating.
Warm, moist air rises at the equator, creating a zone of low pressure known as the Intertropical Convergence Zone (ITCZ).
As this air ascends, it cools, releasing precipitation and creating the characteristically wet tropics.
The now-dry air then flows poleward, eventually descending around 30 degrees latitude.
This descending air creates high-pressure zones associated with deserts and arid regions.
The Hadley Cell's circulation also gives rise to the trade winds, which blow towards the equator.
The Ferrel Cell: Mid-Latitude Mixing
The Ferrel Cell, situated between 30 and 60 degrees latitude, is a more complex system.
It's driven by the movement of the Hadley and Polar cells, acting as a sort of geographical middleman.
Surface air in the Ferrel Cell flows poleward, drawing warm air from the tropics towards higher latitudes.
This movement is responsible for the westerlies, dominant winds in the mid-latitudes.
The Ferrel Cell plays a critical role in the formation of mid-latitude cyclones and weather systems.
The Polar Cell: Arctic Influence
The Polar Cell, located at the poles, is driven by the intense cooling of air in these regions.
Cold, dense air descends at the poles, creating a high-pressure zone.
This air then flows equatorward along the surface, picking up moisture and warmth.
Eventually, the air rises at around 60 degrees latitude, creating a zone of low pressure.
The Polar Cell’s influence is largely confined to the high latitudes.
Polar easterlies result from the cell's circulation.
The Intertropical Convergence Zone (ITCZ): A Hub of Uplift
The Intertropical Convergence Zone (ITCZ) is a critical region where air masses converge near the equator.
This area experiences intense convective uplift, resulting in frequent thunderstorms and heavy rainfall.
The ITCZ is not static, it migrates north and south seasonally, following the sun's path.
This migration significantly impacts rainfall patterns across the tropics.
Resulting Wind Patterns: Global Circulation
The atmospheric convection cells give rise to distinct global wind patterns. These winds play a crucial role in redistributing heat and moisture, significantly influencing regional climates.
The trade winds, westerlies, and polar easterlies are all direct consequences of the circulation within these cells.
Weather Patterns and Cloud Formation
Atmospheric convection plays a direct role in the formation of clouds and precipitation.
As warm, moist air rises, it cools, leading to condensation and cloud formation.
Cumulus clouds are a prime example of clouds formed through convective uplift.
Under the right conditions, this can lead to heavy showers and thunderstorms.
Sea Breeze and Land Breeze: Coastal Convection
Convection also drives localized weather phenomena, such as sea breezes and land breezes.
During the day, land heats up faster than the sea, causing air to rise over land and draw cooler air from the sea, creating a sea breeze.
At night, the opposite occurs; the land cools faster, causing air to sink and flow towards the warmer sea, generating a land breeze.
Fronts: Convective Boundaries
Fronts, the boundaries between air masses of differing temperatures and densities, are intimately connected to atmospheric convection.
The interaction of warm and cold air masses can lead to significant convective activity, often resulting in the formation of storms and precipitation.
The types of fronts (cold, warm, occluded, stationary) depend on the direction and intensity of the air mass movement, impacting the severity and type of weather experienced.
Oceanic Convection: Distributing Heat Across the Globe
Following our exploration of atmospheric convection, it is crucial to examine the oceanic realm and the role convection plays in the world's oceans. The ocean serves as a vast reservoir of thermal energy, and convection within this medium acts as a critical mechanism for its distribution. From localized currents to global-scale circulation patterns, oceanic convection is fundamental to understanding climate regulation and marine ecosystems.
The Ocean as a Convective Heat Engine
The ocean's capacity to absorb and store heat is significantly greater than that of the atmosphere. This immense thermal inertia allows the ocean to moderate global temperatures, preventing extreme fluctuations. Convection facilitates this process by vertically mixing water masses, distributing heat from the surface to deeper layers, and vice versa.
This vertical mixing is not uniform. It's driven by differences in density, which are primarily influenced by temperature and salinity. Warmer, less saline water is less dense and tends to remain at the surface. Colder, more saline water is denser and sinks. This density stratification sets the stage for convective overturning.
Ocean Gyres: Localized Heat Redistribution
Ocean gyres are large-scale, circular currents that dominate the surface circulation patterns of the major ocean basins. These gyres are driven by a combination of wind patterns, the Earth's rotation (Coriolis effect), and continental landmasses.
While primarily wind-driven, gyres also play a significant role in heat redistribution. Warm waters from the equatorial regions are transported poleward along the western boundaries of the gyres, moderating temperatures in higher latitudes. Conversely, colder waters from polar regions are transported equatorward along the eastern boundaries.
Examples include the Gulf Stream in the North Atlantic, which carries warm water towards Europe, and the California Current in the North Pacific, which brings cold water southward along the western coast of North America. These currents have profound impacts on regional climates and marine ecosystems.
Thermohaline Circulation: A Global Conveyor Belt
Thermohaline circulation, also known as the Meridional Overturning Circulation (MOC), is a global-scale system of ocean currents driven by differences in temperature (thermo) and salinity (haline). This circulation acts as a vast conveyor belt, transporting heat, nutrients, and carbon dioxide around the world.
The process begins in the North Atlantic, where cold, salty water becomes dense enough to sink to the ocean floor. This sinking water forms the North Atlantic Deep Water (NADW), which then flows southward along the bottom of the Atlantic Ocean. This deep water eventually reaches the Southern Ocean, where it mixes with other water masses and upwells to the surface.
As the water travels along the surface, it warms and becomes less saline. Eventually, it returns to the North Atlantic, completing the cycle. The thermohaline circulation plays a crucial role in regulating global climate by redistributing heat from the tropics to the poles. Any disruption to this circulation, such as the melting of Arctic ice, could have significant consequences for global climate patterns.
Upwelling and Downwelling: Vertical Transport Mechanisms
Upwelling and downwelling are localized vertical movements of water that are also driven by convection and wind patterns. These processes have significant implications for nutrient distribution and marine productivity.
Upwelling
Upwelling occurs when deep, cold, nutrient-rich water rises to the surface. This process is often driven by winds that push surface water away from a coastline, allowing deeper water to replace it. Upwelling brings essential nutrients to the surface, fueling phytoplankton growth and supporting complex marine food webs. Coastal upwelling zones are among the most productive ecosystems in the world.
Downwelling
Downwelling, conversely, occurs when surface water sinks to deeper layers. This process is often driven by converging currents or changes in water density. Downwelling transports oxygen and carbon dioxide to the deep ocean, playing a role in carbon sequestration. However, it can also suppress nutrient availability in surface waters.
Following our exploration of oceanic convection, it's vital to consider the sophisticated technologies and instruments that enable scientists to observe, measure, and model these complex convective processes.
Tools and Technologies: Observing and Modeling Convection
Understanding convection, in both the atmosphere and the oceans, requires a multi-faceted approach. Direct observation alone is insufficient to grasp the scale and complexity of these phenomena. Therefore, scientists rely on a range of tools and technologies, from sophisticated numerical models to specialized instruments, to probe the inner workings of convective systems.
Weather and Climate Models: Simulating Reality
Weather and climate models are central to understanding and predicting convective processes. These models use complex mathematical equations to simulate the behavior of the atmosphere and oceans.
By incorporating data from various sources, such as satellites and surface observations, these models can provide valuable insights into the dynamics of convection.
Weather models, used for short-term forecasting, focus on simulating atmospheric conditions over days or weeks. They can forecast severe weather events driven by convection, like thunderstorms and hurricanes.
Climate models, used for long-term projections, simulate the Earth's climate system over decades or centuries.
These simulations enable researchers to understand how changes in greenhouse gas concentrations impact global convective patterns. A significant challenge lies in accurately representing the small-scale processes that drive convection within these models. This necessitates continuous refinement of the models' resolution and parameterizations.
Satellites: Eyes in the Sky
Satellites play a pivotal role in providing observational data for weather and ocean monitoring.
Equipped with advanced sensors, satellites can measure various parameters. These include temperature, humidity, wind speed, and sea surface height.
This data is essential for tracking the evolution of convective systems.
Geostationary satellites provide continuous monitoring of specific regions, capturing the dynamic nature of atmospheric convection.
Polar-orbiting satellites offer global coverage, providing comprehensive data on ocean currents and sea surface temperatures that influence oceanic convection. Satellite-based observations are particularly valuable in remote areas, where ground-based measurements are scarce.
Radiosondes: Probing the Atmosphere
Radiosondes, or weather balloons, are indispensable tools for measuring atmospheric conditions. Launched into the atmosphere, these balloons carry instruments that measure temperature, humidity, pressure, and wind speed as they ascend.
The data collected by radiosondes provides a vertical profile of the atmosphere. This is crucial for understanding the stability and potential for convective development. Radiosonde data is also used to validate and improve weather models.
The relatively low cost of radiosondes allows for frequent launches, providing a continuous stream of atmospheric data.
Surface Instruments: Ground-Level Observations
Anemometers, thermometers, and barometers are essential instruments for gathering data related to convective heat transfer at the Earth's surface.
Anemometers measure wind speed and direction.
Thermometers measure air and surface temperature.
Barometers measure atmospheric pressure.
These instruments provide valuable information about the conditions that favor or inhibit convective development. Networks of surface instruments are used to monitor weather conditions across vast areas. This information is essential for nowcasting and short-term forecasting.
Doppler Radar: Detecting Precipitation
Doppler radar is a powerful tool for detecting precipitation intensity and movement.
By emitting radio waves and analyzing the reflected signals, Doppler radar can determine the location, intensity, and direction of precipitation.
This information is critical for tracking severe weather events. These include thunderstorms, tornadoes, and heavy rainfall.
Doppler radar can also measure wind speeds within storms, providing valuable insights into the dynamics of convective systems. The ability to detect and track precipitation in real-time makes Doppler radar an indispensable tool for weather forecasting and public safety.
Video: Convection Cells: Weather, Ocean Currents Explained
FAQs: Convection Cells Explained
What is the driving force behind convection cells?
Heat energy is the primary driver. When a fluid (like air or water) is heated, it becomes less dense and rises. Cooler, denser fluid then sinks to replace it, creating a circular motion. This continuous cycle of rising and sinking fluids establishes convection cells.
How do convection cells impact global weather patterns?
Atmospheric convection cells redistribute heat around the Earth. Warm, moist air rises at the equator, cools and releases rain, then descends at higher latitudes. These cells, such as the Hadley, Ferrel, and Polar cells, directly influence prevailing winds and precipitation zones, thus shaping global weather patterns.
Do convection cells exist in the ocean, too?
Yes, ocean currents are also driven by convection cells. Differences in temperature and salinity create density variations in seawater. Warmer, less salty water rises, while colder, saltier water sinks, driving large-scale ocean circulation patterns via these convection cells.
What happens to air as it rises within a convection cell?
As air rises within a convection cell, it expands and cools due to decreasing atmospheric pressure. This cooling can lead to condensation of water vapor, forming clouds and potentially leading to precipitation. The rising air then eventually cools enough to sink again, completing the convection cells cycle.
So, the next time you're watching clouds bubble up on a sunny day or hear about ocean currents shifting, remember those invisible powerhouses: convection cells. They're a fundamental force shaping our planet's weather and climate, and understanding them helps us appreciate the dynamic, ever-changing world around us. Pretty cool, right?