Cells to Organs: How Does This Body Magic Happen?!
The remarkable journey from single cells to intricately functioning organs, a process heavily studied in developmental biology, truly exemplifies the body's magic. Stem cells, the foundation of this process, possess the incredible ability to differentiate and specialize, a concept explored by researchers worldwide, including at institutions like the National Institutes of Health (NIH). This transformation involves complex signaling pathways and cellular interactions, meticulously orchestrated to build specialized tissues and then fully functioning cells to organs. Understanding this process is crucial for advancing regenerative medicine and treating diseases impacting organ systems.
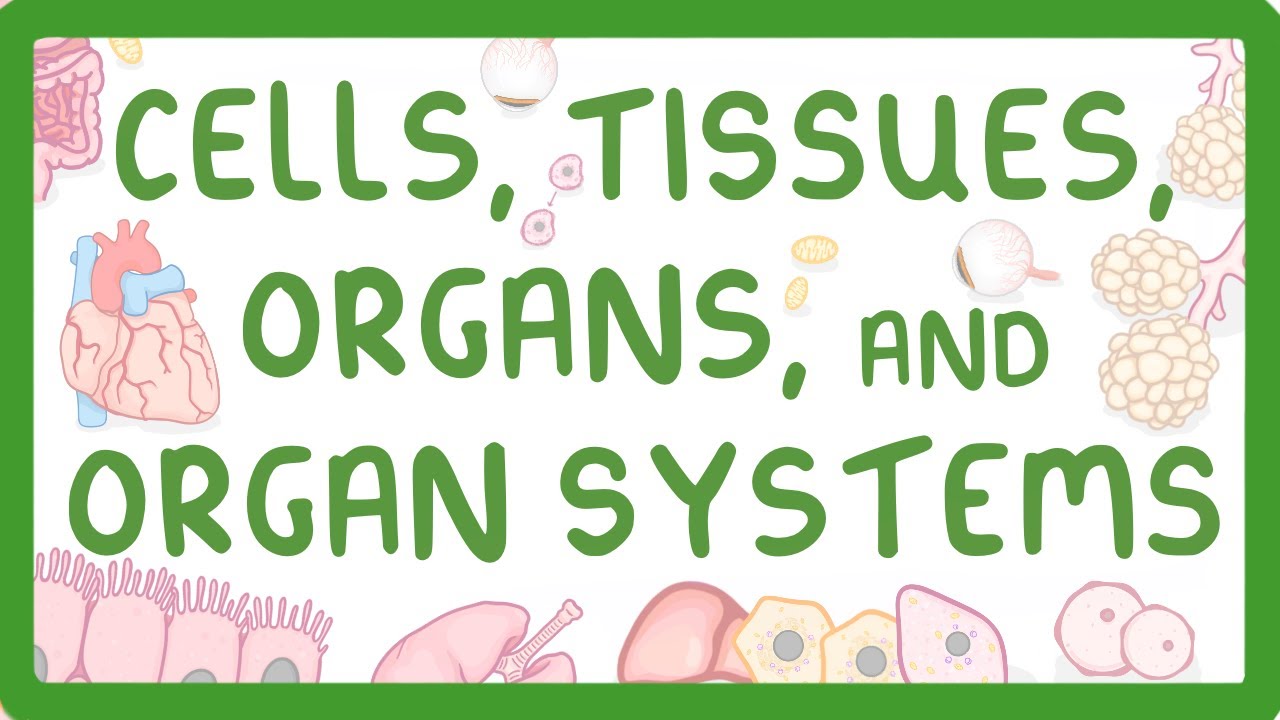
Image taken from the YouTube channel Cognito , from the video titled GCSE Biology - Levels of Organisation - Cells, Tissues, Organs and Organ Systems .
Have you ever stopped to truly consider the sheer complexity of the human body? A single, microscopic cell, barely visible to the naked eye, embarks on a journey of astonishing transformation. It divides, multiplies, and differentiates into trillions of specialized cells. These cells, remarkably, collaborate to construct the intricate architecture of tissues and organs that define our very existence.
This begs the question: how does this seemingly impossible feat of biological engineering occur? How does a single entity orchestrate such an elaborate and coordinated process?
From Single Cell to Complex Organism
The journey begins with a single, totipotent cell, capable of giving rise to any cell type in the body. Through a tightly regulated process of cell division and differentiation, this initial cell proliferates into a vast army of specialized cells. These cells then self-organize into distinct tissues, which, in turn, assemble into complex organs, each performing a unique and vital function.
The Players: Cells, Tissues, and Organs
To understand this remarkable transformation, it’s crucial to define the key players:
-
Cells: The fundamental units of life, responsible for carrying out all essential biological processes.
-
Tissues: Organized groups of cells that work together to perform a specific function.
-
Organs: Complex structures composed of two or more different tissues, working in harmony to execute a specific set of tasks.
Exploring the Cellular Symphony
This article will explore the fascinating world of cellular collaboration, differentiation, and organization. We will delve into the intricate mechanisms that govern how cells communicate, coordinate their activities, and ultimately create the complex structures that comprise the human body. Understanding these processes is not only essential for appreciating the marvels of biological engineering, but also for gaining insights into the origins and treatment of various diseases.
Have you ever stopped to truly consider the sheer complexity of the human body? A single, microscopic cell, barely visible to the naked eye, embarks on a journey of astonishing transformation. It divides, multiplies, and differentiates into trillions of specialized cells. These cells, remarkably, collaborate to construct the intricate architecture of tissues and organs that define our very existence.
This begs the question: how does this seemingly impossible feat of biological engineering occur? How does a single entity orchestrate such an elaborate and coordinated process?
From Single Cell to Complex Organism The journey begins with a single, totipotent cell, capable of giving rise to any cell type in the body. Through a tightly regulated process of cell division and differentiation, this initial cell proliferates into a vast army of specialized cells. These cells then self-organize into distinct tissues, which, in turn, assemble into complex organs, each performing a unique and vital function.
The Players: Cells, Tissues, and Organs To understand this remarkable transformation, it’s crucial to define the key players:
Cells: The fundamental units of life, responsible for carrying out all essential biological processes.
Tissues: Organized groups of cells that work together to perform a specific function.
Organs: Complex structures composed of two or more different tissues, working in harmony to execute a specific set of tasks.
Exploring the Cellular Symphony This article will explore the fascinating world of cellular collaboration, differentiation, and organization. We will delve into the intricate mechanisms that govern how cells communicate, coordinate their activities, and, as we'll discuss in this section, form the very foundation of our being.
Cells: The Fundamental Building Blocks and Their Specialized Roles
If the body is a magnificent city, then cells are its individual bricks, each essential for the integrity and function of the whole. But unlike identical bricks, cells come in a vast array of forms, each expertly tailored for a specific purpose. This section will explore the fascinating world of cells, their differentiation, and how they organize to form the tissues that construct our organs.
Defining the Cell: The Basic Unit of Life
At its core, the cell is the smallest unit of life capable of independently performing life functions. Enclosed by a membrane, a cell contains a complex internal environment where biochemical reactions essential for growth, metabolism, and reproduction take place.
From energy production to waste removal, cells orchestrate a symphony of processes to maintain their own existence and contribute to the well-being of the organism. It is this remarkable self-sufficiency that truly defines the cell as the fundamental building block of life.
Cell Differentiation: From Blank Slate to Specialized Function
Imagine a construction site where every worker initially possesses the potential to perform any task. This is analogous to the early stages of cell development. Through a remarkable process called cell differentiation, cells commit to a specific fate, developing unique structures and functions.
This process allows cells with the same DNA to become vastly different from each other. One cell may become a muscle cell, optimized for contraction; another, a nerve cell, designed for rapid communication.
Factors Influencing Differentiation
Cell differentiation is controlled by a complex interplay of factors, including:
- Gene Expression: Different genes are activated or deactivated, leading to the production of specific proteins.
- Signaling Molecules: Chemical signals from neighboring cells influence gene expression and cellular fate.
- Environmental Cues: Factors such as temperature and nutrient availability can also impact differentiation.
Examples of Specialized Cells
- Muscle Cells (Myocytes): Elongated cells containing contractile proteins, enabling movement.
- Nerve Cells (Neurons): Cells with specialized extensions for transmitting electrical signals.
- Epithelial Cells: Cells that form protective barriers, such as skin and the lining of organs.
The Four Primary Tissue Types: A Foundation of Biological Architecture
Cells don't operate in isolation. Instead, they organize themselves into tissues, groups of similar cells performing a common function. There are four primary tissue types in the human body: epithelial, connective, muscle, and nervous tissue.
Each plays a critical role in the construction and function of our organs.
Epithelial Tissue: The Body's Protective Shield
Epithelial tissue covers surfaces, lining organs and forming glands. Its functions include protection, secretion, absorption, and excretion.
- Structure: Closely packed cells forming a continuous sheet.
- Examples: Skin, lining of the digestive tract, and glands.
- Function: Protects underlying tissues, secretes hormones and enzymes, absorbs nutrients, and excretes waste products.
Connective Tissue: The Support Network
Connective tissue provides support, connects other tissues, and transports substances throughout the body.
- Structure: Cells scattered within an extracellular matrix (ECM) composed of protein fibers and ground substance.
- Examples: Bone, cartilage, blood, and adipose tissue.
- Function: Supports and connects tissues, protects organs, transports nutrients and waste, and stores energy.
Muscle Tissue: The Movers and Shakers
Muscle tissue is responsible for movement. There are three types of muscle tissue: skeletal, smooth, and cardiac.
- Structure: Cells containing contractile proteins (actin and myosin).
- Examples: Skeletal muscles (attached to bones), smooth muscles (lining internal organs), and cardiac muscle (heart).
- Function: Enables movement, maintains posture, and pumps blood.
Nervous Tissue: The Body's Communication Network
Nervous tissue is responsible for communication and control.
- Structure: Cells called neurons and glial cells. Neurons transmit electrical signals, while glial cells support and protect neurons.
- Examples: Brain, spinal cord, and nerves.
- Function: Transmits electrical signals, coordinates body functions, and enables sensation, thought, and memory.
Tissues Contributing to Organ Formation
The four tissue types rarely act in isolation. Rather, they work together to construct the complex structures we call organs.
For instance, the stomach contains all four tissue types:
- Epithelial tissue lines the stomach, protecting it from the harsh acidic environment.
- Connective tissue provides support and structure.
- Muscle tissue contracts to mix food.
- Nervous tissue controls muscle contractions and regulates secretions.
Each tissue plays a critical role in the stomach's function of digesting food.
The arrangement and interaction of these tissues are crucial for the proper functioning of each organ, highlighting the remarkable integration of biological structures. It is through this intricate orchestration that the body achieves its full potential.
Have you ever stopped to truly consider the sheer complexity of the human body? A single, microscopic cell, barely visible to the naked eye, embarks on a journey of astonishing transformation. It divides, multiplies, and differentiates into trillions of specialized cells. These cells, remarkably, collaborate to construct the intricate architecture of tissues and organs that define our very existence.
This begs the question: how does this seemingly impossible feat of biological engineering occur? How does a single entity orchestrate such an elaborate and coordinated process?
The answer lies in the orchestrated interplay of cells, tissues, and organs. Having understood their individual roles, it’s time to explore how these components unite to form the functional units we know as organs, the body’s workhorses.
From Tissues to Organs: A Structural and Functional Symphony
An organ is not merely a collection of cells or a random assortment of tissues. Instead, it's a highly organized, integrated structure composed of at least two different tissue types working in perfect synchrony. This collaboration enables the organ to perform a specific, complex function essential for maintaining life.
Think of it as a musical ensemble. Individual musicians (tissues), each with their unique instrument (cells), come together to create a harmonious melody (organ function). Each part is critical, and the overall effect is greater than the sum of its parts.
Defining the Organ: More Than Just a Sum
Organs are defined by their unique combination of tissue types, their distinct three-dimensional architecture, and the specific function they perform within the body.
For instance, the stomach contains epithelial tissue for protection and secretion, connective tissue for support, muscle tissue for contraction, and nervous tissue for regulation.
It's the precise arrangement and interaction of these tissues that allow the stomach to churn food and initiate digestion.
The Body's Ensemble: A Glimpse at Key Organs
The human body boasts a remarkable variety of organs, each meticulously designed to fulfill a specific role. A few prominent examples include:
-
Heart: The tireless pump, composed primarily of cardiac muscle, responsible for circulating blood throughout the body.
-
Lungs: Spongy organs filled with tiny air sacs (alveoli) that facilitate gas exchange, taking in oxygen and releasing carbon dioxide.
-
Liver: A metabolic powerhouse, performing hundreds of functions, from filtering blood and producing bile to storing nutrients and detoxifying harmful substances.
-
Kidneys: Essential for maintaining fluid and electrolyte balance, filtering waste products from the blood and producing urine.
-
Brain: The command center of the body, responsible for thought, emotion, movement, and countless other functions. A complex network of neurons and supporting cells.
Histology: Unveiling Organ Structure at a Microscopic Level
Histology, the study of tissues at a microscopic level, is instrumental in understanding organ structure and how that structure relates to its function. By examining tissue samples under a microscope, histologists can identify different cell types, observe their arrangement, and detect any abnormalities.
This detailed analysis provides crucial insights into how an organ functions normally and how disease can disrupt that function.
For example, examining a lung tissue sample under a microscope can reveal damage caused by smoking or disease, allowing for a more accurate diagnosis and treatment plan.
Deeper Dive: Exploring Specific Organs
Let's take a closer look at the structure and function of a few key organs:
The Heart: A Symphony of Contraction
The heart, a muscular organ roughly the size of a fist, is the central component of the circulatory system. Its primary function is to pump blood throughout the body, delivering oxygen and nutrients to tissues and removing waste products.
The heart is composed of three layers: the epicardium (outer layer), the myocardium (muscular middle layer), and the endocardium (inner lining). The myocardium, composed of specialized cardiac muscle cells, is responsible for the heart's powerful contractions.
The heart also contains four chambers: two atria (upper chambers) and two ventricles (lower chambers). Valves between these chambers ensure that blood flows in only one direction.
The Lungs: The Breath of Life
The lungs are paired, spongy organs located in the chest cavity. Their primary function is to facilitate gas exchange, allowing oxygen to enter the bloodstream and carbon dioxide to be removed.
Air enters the lungs through the trachea, which branches into two main bronchi, one for each lung. These bronchi further divide into smaller and smaller airways called bronchioles, which eventually terminate in tiny air sacs called alveoli.
The alveoli are surrounded by a dense network of capillaries, tiny blood vessels where gas exchange occurs. The enormous surface area of the alveoli, approximately the size of a tennis court, maximizes the efficiency of gas exchange.
The Liver: The Body's Chemical Factory
The liver, the largest internal organ, performs a vast array of functions essential for life. It filters blood, removes toxins, produces bile (which aids in digestion), stores nutrients, and synthesizes proteins.
The liver is composed of specialized cells called hepatocytes, arranged in hexagonal units called lobules. These lobules are surrounded by blood vessels and bile ducts, which facilitate the liver's diverse functions.
The liver's remarkable regenerative capacity allows it to recover from significant damage.
Orchestrating Development: Cell Communication, Morphogenesis, and the Extracellular Matrix (ECM)
The journey from a single fertilized egg to a complex, functioning organism is a ballet of cellular activity, a meticulously choreographed sequence of events that unfolds with astonishing precision. But who directs this biological performance? The answer lies in a multifaceted system involving constant communication between cells, the dynamic process of morphogenesis, and the supportive framework provided by the extracellular matrix.
These three elements work in concert to sculpt tissues, mold organs, and ultimately bring a living being into existence. Understanding their individual contributions, and how they intertwine, is key to unlocking the secrets of developmental biology.
The Symphony of Cell Communication
Cells are not isolated entities operating in a vacuum. They are social beings, constantly exchanging information to coordinate their actions and ensure the proper execution of developmental programs. Cell communication is the fundamental language of life, allowing cells to sense their environment, respond to signals, and influence the behavior of their neighbors.
This communication occurs through a variety of mechanisms, including:
- Direct Contact: Cells can directly interact with each other through specialized junctions, allowing for the passage of signaling molecules or the transmission of mechanical forces.
- Chemical Signaling: Cells secrete signaling molecules, such as growth factors, hormones, and neurotransmitters, that bind to receptors on other cells, triggering a cascade of intracellular events.
- Electrical Signaling: Some cells, particularly neurons and muscle cells, communicate through electrical signals, allowing for rapid and long-range communication.
These signals act as instructions, guiding cells to divide, differentiate, migrate, or even undergo programmed cell death (apoptosis). Without this constant flow of information, development would quickly descend into chaos.
Morphogenesis: Sculpting Form from Chaos
Morphogenesis is the process by which tissues and organs acquire their specific shapes and structures during development. It's not simply about cells growing in number, but about their coordinated movement, rearrangement, and differentiation to create complex three-dimensional forms.
Think of a sculptor meticulously shaping a block of clay, or a team of architects erecting a building.
Morphogenesis relies on several key mechanisms:
- Cell Proliferation: Controlled cell division is essential for increasing the size of tissues and organs.
- Cell Migration: Cells must migrate to specific locations within the developing embryo to form the correct structures.
- Cell Shape Changes: Cells alter their shapes, becoming elongated, flattened, or polarized, to contribute to tissue architecture.
- Apoptosis (Programmed Cell Death): Selective cell death is crucial for removing unwanted cells and sculpting tissues.
These processes are tightly regulated by cell communication and influenced by the surrounding environment, including the extracellular matrix.
The Extracellular Matrix: More Than Just Scaffolding
The extracellular matrix (ECM) is a complex network of proteins and carbohydrates that surrounds cells in tissues and organs. It's often thought of as a structural support system, providing a framework for cells to adhere to and maintain their shape.
However, the ECM is far more than just scaffolding. It plays a crucial role in regulating cell behavior, influencing cell growth, differentiation, migration, and survival.
The ECM exerts its influence through several mechanisms:
- Providing Structural Support: The ECM provides mechanical support and tensile strength to tissues and organs.
- Regulating Cell Adhesion: Cells adhere to the ECM through specialized receptors, allowing them to sense their environment and receive signals.
- Presenting Growth Factors: The ECM can bind and present growth factors to cells, influencing their proliferation and differentiation.
- Guiding Cell Migration: The ECM provides pathways for cells to migrate along during development and tissue repair.
The composition and organization of the ECM vary depending on the tissue and developmental stage, reflecting its dynamic role in shaping the developing embryo. Understanding the intricate interplay between cells and the ECM is essential for unraveling the mysteries of morphogenesis.
In essence, cell communication, morphogenesis, and the ECM are not separate entities, but rather interconnected components of a sophisticated developmental system. They work together in a coordinated fashion to orchestrate the formation of a complex, functioning organism, highlighting the remarkable precision and elegance of biological processes.
Cells communicate, tissues form, and organs arise. But the story doesn't end there. These individual organs are not solitary actors; they are members of a larger ensemble, working in concert to sustain life itself.
Organ Systems: A Collaborative Network for Life
Organs, remarkable in their individual complexity, truly shine when viewed as integral components of larger, interconnected organ systems. These systems are teams of organs that collaborate to perform complex, life-sustaining functions. Think of it as the body's version of specialized departments within a highly efficient company, each contributing to the overall mission of survival and well-being.
The Body's Orchestrated Teams
These organ systems don't operate in isolation. They are intricately linked, constantly communicating and coordinating their activities to maintain the body's delicate internal balance. Understanding these systems and their interdependencies is crucial to grasping the holistic nature of human physiology.
Core Organ Systems and Their Functions
Let's explore some of the key players in this biological symphony:
The Circulatory System: The Body's Highway
Imagine a vast network of roads and highways crisscrossing a bustling city. That's essentially what the circulatory system is: the body's transportation network.
The heart, the powerful engine at the center, pumps blood through a complex network of vessels: arteries, veins, and capillaries. This system delivers oxygen, nutrients, hormones, and immune cells to every corner of the body. It also removes waste products like carbon dioxide, ensuring that cells receive what they need and are cleansed of harmful byproducts.
Without this constant circulation, cells would quickly suffocate and starve, leading to rapid organ failure.
The Respiratory System: The Breath of Life
The respiratory system is responsible for the vital exchange of gases: bringing in the oxygen we need to survive and expelling the carbon dioxide that is a waste product of cellular respiration.
The lungs, with their intricate network of alveoli, are the primary site of this exchange. Air travels through the airways, including the trachea and bronchi, to reach these tiny air sacs where oxygen diffuses into the bloodstream.
The respiratory system works in close coordination with the circulatory system to ensure that oxygen is efficiently delivered to the tissues and carbon dioxide is removed.
The Digestive System: Fueling the Body
Our bodies require energy to perform all of its tasks. The digestive system breaks down the food we eat into smaller molecules that can be absorbed into the bloodstream and used for energy, growth, and repair.
From the mouth, where digestion begins with chewing and saliva, food travels through the esophagus to the stomach, where it is further broken down by acids and enzymes. The small intestine is the primary site of nutrient absorption, while the large intestine absorbs water and eliminates solid waste.
The liver, pancreas, and gallbladder also play critical roles in digestion, producing enzymes and other substances that aid in the breakdown and absorption of nutrients.
Other Essential Systems
Of course, these are just a few examples. The body also relies on the nervous system for rapid communication, the endocrine system for hormone regulation, the immune system for defense against pathogens, the skeletal and muscular systems for support and movement, the urinary system for waste removal, and the reproductive system for procreation.
Each system plays a vital and irreplaceable role in maintaining the body's overall health and well-being.
These systems exemplify the interdependent nature of life. They work together in a seamless, coordinated fashion to ensure that every cell, tissue, and organ receives what it needs to function optimally.
Cells communicate, tissues form, and organs arise. But the story doesn't end there. These individual organs are not solitary actors; they are members of a larger ensemble, working in concert to sustain life itself.
The collaborative efforts of organ systems allow the body to operate like a finely tuned machine. But what orchestrates this symphony of biological processes? The answer lies in the concept of homeostasis.
Homeostasis: The Body's Internal Balancing Act
Life, in all its complexity, depends on maintaining a stable internal environment. This dynamic equilibrium is known as homeostasis.
It’s the body's remarkable ability to regulate its internal conditions, such as temperature, pH, and nutrient levels, despite fluctuations in the external environment.
Think of it as a biological thermostat, constantly adjusting and fine-tuning to keep everything within a narrow, optimal range.
This internal consistency is essential for cellular function, as cells can only operate efficiently within specific parameters.
The Essence of Stability: Defining Homeostasis
At its core, homeostasis is about maintaining balance.
It's the body's way of saying, "Even though the world outside is constantly changing, inside, we're going to keep things just right."
This involves a complex interplay of physiological processes, working in concert to counteract any disturbances that threaten to disrupt the internal equilibrium.
Without homeostasis, cells would struggle to function, leading to illness and ultimately, death.
The Symphony of Systems: Organ Systems in Concert
Achieving homeostasis is not the responsibility of a single organ or system. It requires the coordinated effort of multiple systems working together.
Each system plays a crucial role in maintaining specific aspects of the internal environment.
Let's consider a few examples:
-
The Respiratory System: Works in tandem with the circulatory system to ensure the bloodstream has proper oxygen and is free of excessive carbon dioxide, maintaining blood pH.
-
The Digestive System: Extracts essential nutrients from food, delivering them to the circulatory system for distribution throughout the body, maintaining the right levels of glucose, amino acids, and fats.
-
The Urinary System: Works with the circulatory system to filter waste products from the blood and regulate fluid and electrolyte balance, ensuring the proper concentration of salts and water in the body.
-
The Endocrine System: Secretes hormones that act as chemical messengers, coordinating the activity of various organs and systems to maintain long-term stability.
Negative Feedback Loops: The Body's Control Mechanism
A key mechanism that the body uses to maintain homeostasis is the negative feedback loop.
This is a self-regulating process where a change in a particular parameter triggers a response that counteracts the initial change, bringing the system back to its set point.
Imagine a thermostat in your home. When the temperature drops below the set point, the heater turns on, raising the temperature.
Once the set point is reached, the heater turns off, preventing the temperature from rising too high.
The body uses similar feedback loops to regulate blood pressure, blood sugar levels, body temperature, and many other vital parameters.
Disruptions and Disease: When Balance is Lost
When the body's homeostatic mechanisms fail, the internal environment can become unstable, leading to illness and disease.
For example, diabetes is a condition characterized by the body's inability to regulate blood sugar levels, resulting in hyperglycemia (high blood sugar).
Similarly, hypertension (high blood pressure) occurs when the body's blood pressure control mechanisms are disrupted, leading to chronically elevated blood pressure.
Understanding how homeostasis works and what can disrupt it is crucial for preventing and treating a wide range of health conditions.
By maintaining this delicate internal balance, our bodies allow cells, tissues, and organs to function optimally.
Cells communicate, tissues form, and organs arise. But the story doesn't end there. These individual organs are not solitary actors; they are members of a larger ensemble, working in concert to sustain life itself. The collaborative efforts of organ systems allow the body to operate like a finely tuned machine. But what orchestrates this symphony of biological processes? The answer lies in the concept of homeostasis.
But even with precise regulation and constant maintenance, the body also relies on a less intuitive, yet equally vital, process: programmed cell death. It may seem counterproductive, but the controlled elimination of cells is as crucial to life as cell growth and division. This process, known as apoptosis, is not a sign of failure but rather an essential act of biological artistry.
Apoptosis: Programmed Cell Death – Sculpting Life
The Necessity of Cellular Self-Sacrifice
Apoptosis, or programmed cell death, is a fundamental process that plays a critical role in the development and maintenance of healthy tissues.
Unlike necrosis, which is a messy and uncontrolled cell death often resulting from injury or infection, apoptosis is a carefully orchestrated event, a cellular suicide mission with specific goals.
It's the body's way of pruning, shaping, and refining its tissues, ensuring that only the fittest and most functional cells remain.
Think of a sculptor meticulously chipping away at a block of marble to reveal a masterpiece.
Apoptosis is the cellular equivalent, removing unwanted or damaged cells to create the final form.
Apoptosis in Development: From Tadpole to Human
One of the most striking examples of apoptosis can be seen during embryonic development. Consider the formation of fingers and toes.
In the early stages of development, the hand and foot resemble paddles.
It is through apoptosis that the cells between the developing digits are eliminated, sculpting the individual fingers and toes we recognize.
Similarly, the development of the nervous system involves a massive overproduction of neurons, followed by the selective elimination of those that fail to make proper connections.
This process ensures that only the functional neural circuits are retained, optimizing the efficiency of the brain.
Apoptosis also plays a crucial role in the development of the immune system, eliminating self-reactive immune cells that could potentially attack the body's own tissues.
Sculpting the Tadpole's Tail
A particularly vivid example of apoptosis is the metamorphosis of a tadpole into a frog.
As the tadpole transitions into its adult form, its tail is no longer needed.
Through the precise execution of apoptosis, the cells of the tail are broken down and recycled, providing building blocks for the developing limbs and other adult structures.
Apoptosis in Tissue Maintenance: A Constant Renewal
Apoptosis is not just a developmental process; it continues to be essential throughout adult life.
It plays a vital role in maintaining tissue homeostasis by removing old, damaged, or infected cells.
For example, in the lining of the intestine, cells are constantly being shed and replaced.
Apoptosis ensures that these old cells are removed in a controlled manner, preventing inflammation and maintaining the integrity of the gut barrier.
Similarly, in the immune system, apoptosis helps to control the number of immune cells, preventing excessive immune responses that could lead to autoimmune diseases.
When Apoptosis Goes Wrong: Disease Implications
Given its crucial role, it's not surprising that dysregulation of apoptosis is implicated in a wide range of diseases.
Too much apoptosis can lead to neurodegenerative disorders like Alzheimer's and Parkinson's disease, where neurons are prematurely lost.
It can also contribute to autoimmune diseases, where the failure to eliminate self-reactive immune cells leads to attacks on the body's own tissues.
Too little apoptosis, on the other hand, can contribute to cancer, where cells that should have been eliminated continue to grow and divide uncontrollably.
Understanding the intricate mechanisms that regulate apoptosis is therefore crucial for developing new therapies for these diseases.
A Delicate Balance
Apoptosis, therefore, is not simply about cell death. It’s about balance. It is an active and regulated process, essential for sculpting life, maintaining tissue health, and preventing disease.
Its significance highlights the body's remarkable ability to not only create but also to selectively eliminate, demonstrating that sometimes, less truly is more.
Video: Cells to Organs: How Does This Body Magic Happen?!
FAQs About Cells to Organs
Here are some frequently asked questions about how individual cells organize themselves into complex organs. Understanding this process is key to understanding how our bodies function.
How exactly do cells know where to go to form a specific organ?
Cells don't act randomly! They receive signals, both chemical and physical, that guide their movement and behavior. These signals tell cells what to become, where to move, and who to interact with, ensuring they find their correct place within a developing organ. It’s like a precisely choreographed dance.
What's the role of cell differentiation in the formation of organs?
Cell differentiation is crucial. It's the process where a generic cell "decides" to become a specific type of cell, like a muscle cell or a nerve cell. This specialization is directed by gene expression and allows different cells to perform unique functions within the final organ structure. Without differentiation, we wouldn't have the variety of cell types needed to build complex cells to organs.
What happens if the process of cell organization goes wrong during organ development?
Errors in cell organization can lead to birth defects or developmental disorders. Misplaced or improperly differentiated cells can disrupt the organ's structure and function. Research is ongoing to better understand these processes and develop treatments for such conditions, focusing on getting cells to organs correctly.
Is it possible to artificially grow organs from cells in a lab?
Yes, researchers are making strides in creating artificial organs through tissue engineering. This involves providing the right environment and signals to cells to guide them in forming the desired organ structure. This research holds huge potential for treating organ failure and understanding fundamental aspects of how cells to organs are created in the human body.