Calculate % Change in Mass: Step-by-Step Guide
In scientific experiments, accurate measurement of mass is crucial, and the analytical balance serves as the fundamental instrument for this purpose. Understanding how do we calculate % change in mass? allows researchers to interpret data collected during experiments on chemical reactions, where the mass of reactants and products are carefully monitored. This calculation is vital in fields like stoichiometry, where understanding the quantitative relationships between substances is key to predicting reaction outcomes. The precise calculation of percent change in mass is therefore a critical tool in assessing experimental success and validating theoretical models.
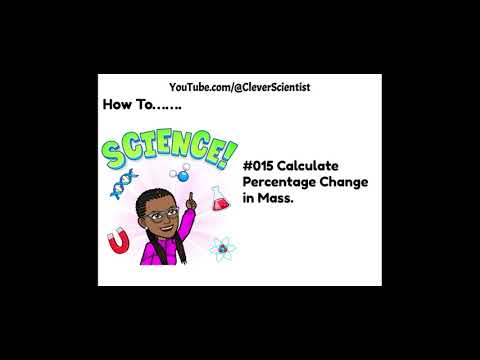
Image taken from the YouTube channel Clever Scientist , from the video titled #015 How To Calculate Percentage Change in Mass. .
Mass, a fundamental property of matter, is a measure of an object's resistance to acceleration. It's an intrinsic characteristic, providing a quantitative measure of inertia. Understanding mass is foundational to numerous scientific principles and practical applications.
Quantifying Mass Variations: Introducing Percentage Change
While absolute mass is crucial, often it's the change in mass that provides critical insights. Percentage change (% Change) is a powerful tool to quantify these variations.
It expresses the relative difference between an initial and final mass as a percentage of the initial mass. This allows for easy comparison of mass changes across different samples or experimental conditions, regardless of the initial mass values.
The Significance of Percentage Change in Mass
Percentage change in mass is not merely a mathematical exercise. It offers valuable insights across diverse scientific and real-world applications.
Scientific Relevance
In chemistry, it helps determine reaction yields and analyze compositional changes. Physics utilizes it to study phenomena involving mass transfer or loss. Biology relies on it for growth studies, osmotic pressure experiments, and metabolic analyses.
Real-World Applications
The applications extend beyond controlled laboratory settings. In manufacturing, it ensures quality control by tracking material loss during production. In medicine, it monitors patient weight changes as indicators of health or disease progression. In environmental science, it helps assess pollution levels and track the effects of climate change on ecosystems.
Understanding percentage change in mass provides a normalized perspective on mass alterations, allowing for meaningful comparisons and informed decision-making in a wide range of disciplines. By grasping its significance, we unlock a powerful analytical tool applicable from the laboratory bench to real-world problem-solving.
Essential Concepts: Initial Mass, Final Mass, and Change in Mass
Mass, a fundamental property of matter, is a measure of an object's resistance to acceleration. It's an intrinsic characteristic, providing a quantitative measure of inertia. Understanding mass is foundational to numerous scientific principles and practical applications.
Quantifying Mass Variations: Introducing Percentage Change
While absolute mass measurements are crucial, often the change in mass is what reveals valuable insights. Percentage change in mass provides a standardized way to express this variation, irrespective of the initial mass value. However, before delving into calculations, understanding the underlying concepts of initial mass, final mass, and change in mass is paramount. These form the very basis for accurately determining percentage change.
Defining Initial Mass: The Starting Point
Initial mass represents the mass of an object or system at the beginning of an observation or experiment. It is the reference point against which all subsequent mass changes are measured.
Accurate determination of the initial mass is absolutely critical. Errors in the initial mass value will propagate through the entire calculation, leading to an inaccurate percentage change. Think of it as setting a proper foundation; a faulty start undermines everything that follows.
For example, when studying the growth of a plant, the initial mass would be the mass of the seed before germination. In a chemical reaction, it would be the mass of the reactants before they begin to react.
Understanding Final Mass: The Endpoint of Measurement
Final mass is the mass of the object or system at the end of the observation or experiment. It represents the mass after a process or change has occurred.
Like initial mass, accurate measurement of the final mass is essential for reliable results. This measurement should be performed with the same level of precision and using the same equipment as the initial mass measurement. Consistency is key.
Continuing our plant growth example, the final mass would be the mass of the plant after a specific period of growth. In the chemical reaction, it would be the mass of the products after the reaction has completed.
Calculating Change in Mass: The Difference That Matters
The change in mass is simply the difference between the final mass and the initial mass. Mathematically, it's expressed as:
Change in Mass = Final Mass - Initial Mass
The change in mass can be either positive or negative. A positive change indicates a mass increase, while a negative change indicates a mass decrease.
The change in mass provides the raw magnitude of the mass variation. However, it doesn't provide context regarding the relative significance of that change. A change of 1 gram is far more significant if the initial mass was 2 grams than if the initial mass was 100 grams. This is where percentage change becomes essential.
By understanding the initial mass, final mass, and change in mass, scientists and researchers can accurately quantify and interpret mass variations in a wide range of applications. This understanding lays the groundwork for the percentage change calculation, which offers valuable insight into the significance of mass alteration across diverse scientific domains.
Calculating Percentage Change: A Step-by-Step Guide
Mass, a fundamental property of matter, is a measure of an object's resistance to acceleration. It's an intrinsic characteristic, providing a quantitative measure of inertia. Understanding mass is foundational to numerous scientific principles and practical applications.
Quantifying mass changes accurately is essential in diverse fields. This section provides a detailed walkthrough of calculating percentage change in mass. It will also break down the formula into its core components. This ensures a clear understanding of the underlying mathematical principles.
The Core Formula: Unveiling Percentage Change
The formula for calculating percentage change in mass is:
% Change = [(Final Mass - Initial Mass) / Initial Mass] * 100
This equation essentially captures the relative difference between the final and initial states, expressed as a percentage. Each component plays a pivotal role.
Subtraction: Isolating the Mass Difference
The first step involves subtracting the initial mass from the final mass. This calculation (Final Mass - Initial Mass) yields the change in mass.
The sign of this value is also critically important. A positive value indicates an increase in mass, while a negative value signals a decrease in mass. This change (positive or negative) forms the numerator of our fraction.
Division: Normalizing the Change
The next step involves dividing the change in mass by the initial mass. This division normalizes the change relative to the starting point. This normalization is crucial.
It allows for meaningful comparisons across different scenarios, regardless of the initial mass values. It answers the question, "How significant is this change relative to what we started with?".
Multiplication: Expressing as a Percentage
Multiplying the result of the division by 100 converts the decimal value into a percentage. This percentage represents the change in mass relative to the initial mass.
This conversion to a percentage makes the change easily interpretable and readily comparable. It facilitates clear communication of the magnitude of the mass change.
The Critical Role of Significant Figures
Significant figures are crucial for representing the precision of your measurements. The final answer cannot be more precise than the least precise measurement used in the calculation.
Maintaining the correct number of significant figures ensures that your calculated percentage change accurately reflects the certainty of your experimental data. Rounding appropriately is paramount.
Leveraging Tools: Calculators and Spreadsheets
While the calculation itself is straightforward, the use of calculators or spreadsheets can greatly enhance efficiency. They can also improve accuracy, especially when dealing with complex datasets.
Spreadsheets, in particular, are invaluable for performing percentage change calculations on multiple data points simultaneously. They allow for organized data management and streamlined analysis. They also reduce the likelihood of errors.
Applications Across Scientific Disciplines: From Chemistry to Nutrition
Calculating percentage change in mass finds widespread utility across diverse scientific fields. Its relevance stems from the fundamental nature of mass as a key property of matter. By quantifying how mass changes in different processes, scientists can gain valuable insights. This includes validating theoretical models to optimizing experimental conditions.
Chemistry: Stoichiometry and Reaction Yields
In chemistry, percentage change in mass is critical for understanding chemical reactions and their efficiency. Stoichiometry, the study of quantitative relationships between reactants and products in chemical reactions, relies heavily on precise mass measurements. By determining the initial and final masses of reactants and products, chemists can calculate the percent yield. This serves as a direct indicator of reaction efficiency.
The percent yield is defined as the ratio of the actual yield to the theoretical yield, expressed as a percentage. This calculation depends on mass measurements. Significant discrepancies between the theoretical and actual yields prompt further investigation. This is to identify potential sources of error or incomplete reactions.
Furthermore, understanding mass changes during reactions is essential for balancing chemical equations. It ensures that the law of conservation of mass is upheld.
Physics: Conservation Laws and Experimental Validation
Physics utilizes percentage change in mass to validate fundamental conservation laws. The law of conservation of mass states that mass is neither created nor destroyed in a closed system. It can only be transferred or transformed.
Experiments designed to test this law often involve precise measurements of mass before and after a physical process or interaction. Any significant change in mass, outside the margin of error, challenges the validity of the experimental setup. This could also mean challenging the understanding of the underlying physical principles.
For example, in nuclear physics, mass-energy equivalence (E=mc²) dictates that a small amount of mass can be converted into a tremendous amount of energy, as seen in nuclear reactions.
Measuring the mass difference between reactants and products allows physicists to calculate the energy released or absorbed in these reactions.
Biology: Growth Rates and Osmotic Processes
In biology, percentage change in mass is an essential tool for studying growth rates, osmotic processes, and overall organismal mass changes. Growth rates of organisms or cell cultures are often quantified by measuring the change in mass over time.
This allows scientists to assess the impact of various environmental factors or treatments. It could be nutrients, temperature, or exposure to toxins on biological systems. Osmosis, the movement of water across a semipermeable membrane, can also be studied using percentage change in mass.
By measuring the mass changes of cells or tissues placed in solutions of varying concentrations, biologists can determine the osmotic pressure and understand how water balance is maintained.
Nutrition Science: Tracking Body Mass and Composition
Nutrition science employs percentage change in mass to assess the effectiveness of dietary interventions and exercise programs. Changes in body mass are directly correlated with changes in energy balance. A positive percentage change suggests caloric surplus, while a negative change indicates a caloric deficit.
However, body composition is also important. Changes in mass can reflect changes in muscle mass, fat mass, or water content. Techniques such as bioelectrical impedance analysis or DEXA scans are often used in conjunction with mass measurements to provide a more detailed picture of body composition changes.
Materials Science: Corrosion, Erosion, and Deposition
Materials science relies on percentage change in mass to evaluate the durability and performance of materials under various conditions. Corrosion, erosion, and deposition processes all involve changes in the mass of a material.
By measuring the mass loss due to corrosion or erosion, engineers can assess the material's resistance to degradation. Similarly, measuring the mass gain due to deposition allows scientists to study the formation of thin films or coatings. Understanding these mass changes is crucial for selecting appropriate materials for specific applications. It also helps developing protective strategies to extend the lifespan of structures and components.
Tools and Techniques for Measuring Mass
Calculating percentage change in mass finds widespread utility across diverse scientific fields. Its relevance stems from the fundamental nature of mass as a key property of matter. By quantifying how mass changes in different processes, scientists can gain valuable insights. To ensure these insights are accurate, understanding the tools and techniques for precise mass measurement is paramount.
Balances: The Cornerstone of Mass Measurement
The primary tool for determining mass is the balance, often referred to colloquially as a "scale." These instruments compare the weight of an object against a known standard. Modern balances typically employ electronic sensors to provide a digital readout of the mass.
The accuracy and precision of a balance are crucial factors to consider, depending on the specific application. Different types of balances cater to varying needs, from routine laboratory work to highly sensitive research applications.
Analytical Balances: Precision in Research
For applications demanding the utmost precision, the analytical balance is indispensable. These instruments are designed to measure mass with extremely high accuracy, often to the nearest 0.0001 gram (0.1 mg) or even better.
Analytical balances are commonly used in research laboratories, where precise measurements are critical for accurate data collection and analysis. Their sensitivity necessitates careful operation, often within enclosed environments to minimize the effects of air currents and vibrations.
Triple Beam Balances: A Mechanical Alternative
While electronic balances are now commonplace, the triple beam balance remains a valuable and reliable mechanical option. This type of balance uses a system of weights that slide along three beams to achieve equilibrium.
Triple beam balances are robust and do not require a power source, making them suitable for field work or situations where electricity is unavailable. While not as precise as analytical balances, they offer sufficient accuracy for many general laboratory applications.
Weighing: The Act of Measuring Mass
The act of determining mass using a balance is referred to as weighing. This process involves placing the object of interest on the balance pan and obtaining a stable reading.
However, accurate weighing requires careful attention to detail. Factors such as proper balance calibration, sample preparation, and environmental conditions can all influence the accuracy of the measurement.
The Importance of Tare
Before weighing a sample, it is essential to tare the balance. Taring involves setting the balance reading to zero with an empty container (e.g., a weighing boat or flask) on the pan.
This step effectively subtracts the mass of the container, ensuring that only the mass of the sample is measured. Taring is crucial for accurate determination of the sample's mass, especially when dealing with small quantities.
Minimizing Errors: Calibration, Environment, and Technique
Calculating percentage change in mass finds widespread utility across diverse scientific fields. Its relevance stems from the fundamental nature of mass as a key property of matter. By quantifying how mass changes in different processes, scientists can gain valuable insights. To ensure these insights are accurate, meticulous attention must be paid to minimizing errors in mass measurements.
Measurement errors can arise from a variety of sources, ranging from the instrument itself to the surrounding environment and the technique employed by the operator. Understanding these potential pitfalls and implementing strategies to mitigate them is paramount for reliable scientific results.
The Imperative of Calibration
Calibration is arguably the most critical step in ensuring balance accuracy. A properly calibrated balance provides a reliable baseline for all subsequent measurements.
Calibration involves comparing the balance's readings against known standards of mass. Any discrepancies are then corrected, either automatically by the balance or manually by the user.
Regular calibration, using certified reference weights, is essential to compensate for drift and maintain accuracy over time. The frequency of calibration depends on the balance's usage, the criticality of the measurements, and the manufacturer's recommendations.
Understanding Measurement Error and Uncertainty
It is crucial to acknowledge that no measurement is perfect. All measurements are subject to some degree of error and, therefore, possess inherent uncertainty.
Measurement error can be broadly classified into two categories: systematic errors and random errors. Systematic errors are consistent and predictable, often stemming from instrumental defects or flawed procedures. Random errors, on the other hand, are unpredictable fluctuations that can arise from various factors, such as environmental noise or subjective judgment.
Quantifying and minimizing uncertainty is an integral part of the scientific process. Techniques such as repeated measurements and statistical analysis can be employed to estimate and reduce the impact of random errors.
The Influence of Environmental Conditions
Environmental conditions can significantly impact mass measurements, particularly when dealing with high-precision balances. Temperature fluctuations can cause the balance components to expand or contract, leading to inaccurate readings.
Humidity can also affect measurements, especially for hygroscopic materials that readily absorb moisture from the air. Ideally, mass measurements should be performed in a controlled environment with stable temperature and humidity levels.
Air currents and vibrations can also introduce noise into the measurements. Therefore, it is crucial to place the balance on a stable surface, away from drafts and other sources of disturbance.
Contamination Control: A Matter of Precision
Contamination can be a significant source of error in mass measurements. Even minuscule amounts of foreign material can alter the mass of a sample, leading to inaccurate results.
Proper handling techniques, such as using clean containers and avoiding direct contact with samples, are essential to prevent contamination.
Regular cleaning of the balance and the surrounding workspace is also crucial for maintaining a pristine measurement environment.
Mastering Weighing Techniques
Proper weighing techniques are essential to minimize errors and ensure accurate measurements.
-
Taring: Always tare the balance before weighing a sample. This sets the balance to zero with the weighing container in place, eliminating its mass from the measurement.
-
Centering the Load: Place the sample in the center of the weighing pan. Off-center loading can introduce errors due to variations in the balance's sensitivity across the pan surface.
-
Allowing Stabilization: Wait for the balance to stabilize before recording the measurement. Rushing the process can lead to inaccurate readings due to fluctuations in the display.
-
Consistent Procedures: Adhere to consistent procedures for all measurements. This helps to minimize random errors and improve the reproducibility of the results.
Illustrative Examples: Real-World Scenarios of Mass Change
Calculating percentage change in mass finds widespread utility across diverse scientific fields. Its relevance stems from the fundamental nature of mass as a key property of matter. By quantifying how mass changes in different processes, scientists can gain valuable insights. To ensure these calculations have a tangible connection to the real world, let's explore several illustrative examples. These scenarios demonstrate how mass changes and how we can measure and interpret them.
Dissolving Sugar in Water: Conservation of Mass
One of the most fundamental principles in chemistry is the law of conservation of mass. This law dictates that mass is neither created nor destroyed in ordinary chemical reactions and physical transformations. Dissolving sugar in water offers a simple yet compelling demonstration of this principle.
Before dissolving, you have separate, measurable masses for both the sugar and the water. The sugar is solid, has its weight, and the water is liquid with its own weight.
When sugar dissolves, it disperses evenly throughout the water, forming a homogeneous solution. Measuring the mass of the solution should equal the sum of the initial masses of the sugar and the water. Any deviation could suggest experimental error, such as spillage or incomplete dissolution. This example emphasizes that while the form of the sugar changes, its mass remains constant within the system.
Evaporation: Illustrating Mass Loss
Evaporation provides a clear example of mass loss from a system. Consider a beaker containing a known mass of water. If left exposed to the air, the water will gradually evaporate. The water turns into water vapor.
As water molecules transition from the liquid to the gaseous phase, they escape into the surrounding environment. This results in a decrease in the mass of the water remaining in the beaker.
By carefully measuring the initial and final masses of the beaker and its contents, one can calculate the percentage change in mass due to evaporation.
This example illustrates how phase transitions can directly impact the measurable mass of a substance within a defined system.
Chemical Reactions: Precipitation
Chemical reactions often involve the formation of new substances with different properties and, crucially, different masses.
Precipitation reactions are a prime example. When two aqueous solutions react to form an insoluble solid (the precipitate), the mass of the system changes depending on what is being measured.
For example, if a solution of silver nitrate (AgNO3) is mixed with a solution of sodium chloride (NaCl), a white precipitate of silver chloride (AgCl) forms.
If the precipitate is collected, dried, and weighed, its mass represents the mass of the new substance formed. Comparing this mass to the initial masses of the reactants allows for calculation of percentage yield and assessment of reaction efficiency.
Dehydration: Mass Loss due to Water Removal
Dehydration, the removal of water from a substance, is another common scenario where mass changes are readily observed. This can occur in both living and non-living systems.
Consider a piece of fruit left out in the open air. Over time, the fruit will lose moisture through evaporation, causing it to shrink and become lighter.
The percentage change in mass directly reflects the amount of water lost during the dehydration process. This principle is used in food preservation techniques, such as drying fruits and vegetables, to inhibit microbial growth and extend shelf life.
Corrosion: Change in Mass from Environmental Reactions
Corrosion is the gradual degradation of a material, typically a metal, due to chemical reactions with its environment. A familiar example is the rusting of iron.
When iron reacts with oxygen and water, it forms iron oxide (rust), which has a different chemical composition and mass than the original iron.
The mass of the corroded material will increase due to the incorporation of oxygen and water molecules into the rust. Measuring the initial and final masses of the metal allows for the calculation of the percentage change in mass resulting from the corrosion process.
This is critical in engineering for assessing the long-term integrity of structures and components.
Osmosis: Mass Changes in Cells or Tissues
Osmosis, the movement of water across a semipermeable membrane from a region of high water concentration to a region of low water concentration, can lead to significant mass changes in biological systems.
Consider a cell placed in a hypertonic solution (a solution with a higher solute concentration than the cell's interior). Water will move out of the cell, causing it to shrink and lose mass.
Conversely, a cell placed in a hypotonic solution will gain water, causing it to swell and increase in mass.
By carefully measuring the mass of cells or tissues before and after exposure to different osmotic environments, one can quantify the percentage change in mass and gain insights into the osmotic properties of the system.
This is crucial for understanding cell function, tissue hydration, and the effects of various solutions on living organisms.
Video: Calculate % Change in Mass: Step-by-Step Guide
<h2>Frequently Asked Questions</h2>
<h3>What does a negative % change in mass indicate?</h3>
A negative % change in mass means the final mass is less than the initial mass. In other words, the object lost mass. When we calculate % change in mass and the result is negative, we know there was a decrease.
<h3>Why is calculating % change in mass important in science?</h3>
It allows us to quantify the difference in mass over time or due to a specific process. This quantification is crucial for analyzing experiments, understanding chemical reactions, and observing growth or decay patterns. Knowing how do we calculate % change in mass helps us measure these changes accurately.
<h3>What's the difference between % change in mass and % error?</h3>
% change in mass measures how much the mass of something has increased or decreased. % error compares an experimental value to a true or accepted value. They are different calculations for different purposes. When we calculate % change in mass, we are not necessarily comparing it to a "true" mass.
<h3>What happens if the initial mass is zero when calculating % change in mass?</h3>
If the initial mass is zero, calculating % change in mass is undefined. Division by zero is not possible. You cannot determine a percentage change if there was no initial mass to begin with. Therefore, how do we calculate % change in mass cannot be applied in this scenario.
So, there you have it! Figuring out percentage change in mass doesn't have to be a headache. Just remember the formula, plug in your numbers, and you're golden. Hopefully, this guide cleared up any confusion about how do we calculate % change in mass? Now go forth and conquer those experiments!