Bromine Valence Electrons: A Student's Guide
The understanding of bromine valence electrons is foundational for students venturing into the complexities of chemical bonding, particularly when applying concepts learned in introductory courses like Chemistry 101. Linus Pauling's work on electronegativity scales provides a crucial framework for predicting how bromine, element number 35 on the periodic table, will interact with other elements to form compounds. Moreover, software tools like ChemDraw can visually represent the electron configurations and bonding behavior of bromine, including its valence electrons, enhancing comprehension. The periodic trends, particularly the halogen group properties, dictate that bromine valence electrons influence its reactivity and bonding characteristics.
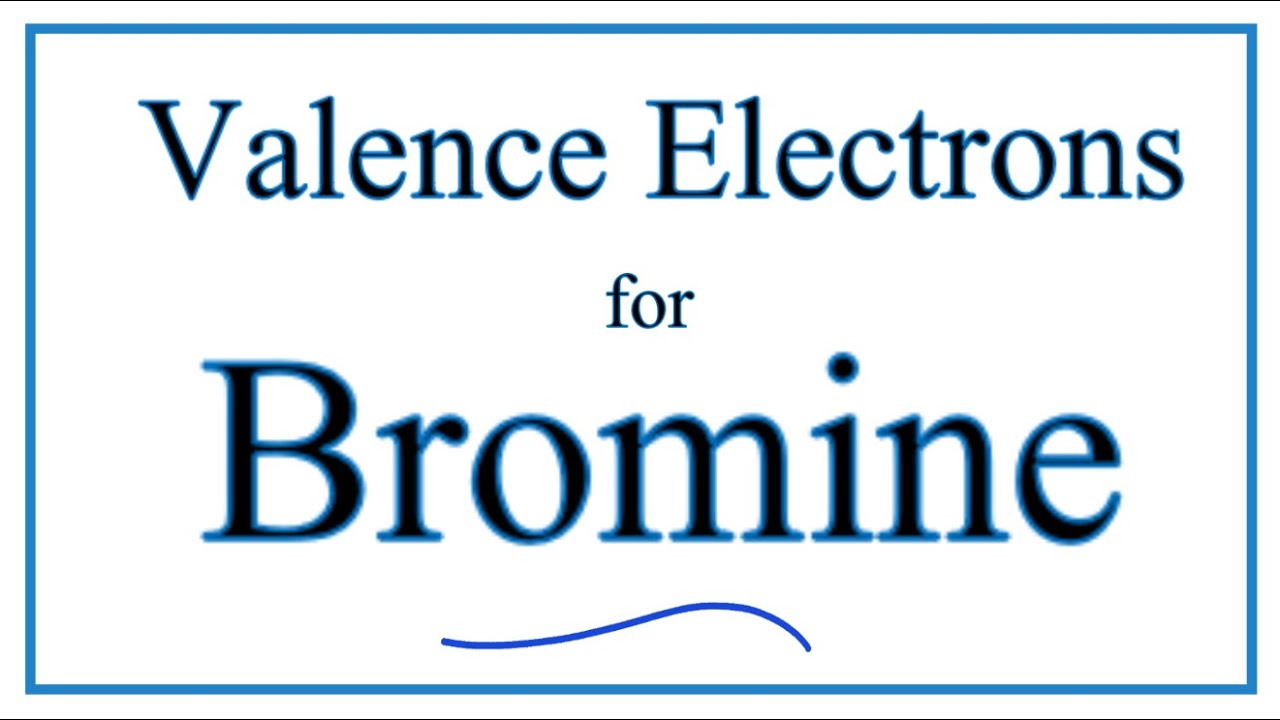
Image taken from the YouTube channel Wayne Breslyn (Dr. B.) , from the video titled How to Find the Valence Electrons for Bromine (Br) .
Bromine, a name that often evokes images of laboratories and complex chemical reactions, is an element of considerable significance in both scientific and industrial realms. This section will serve as an introduction to this fascinating element, exploring its fundamental properties, its place within the periodic table, and the diverse applications that make it indispensable to modern life.
Defining Bromine: Symbol, Atomic Number, and Atomic Weight
Bromine, represented by the symbol Br, occupies a unique position in the periodic table. Its atomic number, 35, signifies that each bromine atom possesses 35 protons within its nucleus. This defining characteristic dictates its chemical behavior and distinguishes it from all other elements.
The standard atomic weight of bromine is approximately 79.904 atomic mass units (amu). This value reflects the average mass of bromine atoms, considering the naturally occurring isotopes of the element.
Discovery and Etymology
The discovery of bromine is attributed to the French chemist Antoine Jérôme Balard in 1826. Balard isolated bromine from seawater, recognizing it as a previously unknown element.
The name "bromine" is derived from the Greek word bromos, meaning "stench" or "bad smell." This etymology aptly describes the element's pungent and irritating odor.
Bromine as a Halogen: Position and Properties
Bromine belongs to Group 17 of the periodic table, a family of elements known as the halogens. These elements, which also include fluorine, chlorine, iodine, and astatine, share similar chemical properties due to their electron configurations.
As a halogen, bromine is characterized by its high reactivity and its tendency to gain one electron to achieve a stable electron configuration. This electron affinity drives its participation in numerous chemical reactions.
Trends within the Halogen Group
Several trends are observed as one descends the halogen group in the periodic table. Electronegativity, which is the measure of an atom's ability to attract electrons in a chemical bond, decreases down the group.
Reactivity also generally decreases from fluorine to iodine, although bromine remains a potent oxidizing agent. The physical state of the halogens transitions from gases (fluorine, chlorine) to a liquid (bromine) to solids (iodine, astatine) at room temperature.
Common Uses and Applications: A Glimpse
Bromine and its compounds find widespread use in various industries. It is a crucial component in the production of flame retardants, which are added to plastics, textiles, and electronics to prevent or slow the spread of fire.
Bromine compounds also play a vital role in the pharmaceutical industry, serving as building blocks in the synthesis of various drugs. Additionally, bromine has applications in agriculture, where it is used as a soil fumigant to control pests and diseases.
These are just a few examples of the many ways in which bromine impacts our lives. Further exploration will reveal the depth and breadth of this element's importance in the modern world.
Atomic Structure and Electron Configuration: Delving into Bromine's Core
Bromine, a name that often evokes images of laboratories and complex chemical reactions, is an element of considerable significance in both scientific and industrial realms. This section will serve as an introduction to this fascinating element, exploring its fundamental properties, its place within the periodic table, and the diverse applications. Before we can understand its reactivity and interactions, we must first examine its atomic structure and electron configuration. Understanding the electron arrangement unlocks the key to predicting its chemical behavior.
Unveiling the Electron Configuration of Bromine
The electron configuration describes the arrangement of electrons within an atom. For bromine, this arrangement dictates how it interacts with other elements.
It provides a roadmap to understanding its bonding capabilities and reactivity. The complete electron configuration of bromine (Br) is 1s² 2s² 2p⁶ 3s² 3p⁶ 4s² 3d¹⁰ 4p⁵.
This notation may seem complex, but it represents the filling of electron shells and subshells, a fundamental principle in atomic theory.
The Aufbau Principle and Orbital Filling
The filling of these orbitals follows the Aufbau principle, which states that electrons first occupy the lowest energy levels available.
This means electrons will first fill the 1s orbital, then the 2s, followed by the 2p, and so on.
The order of filling isn't always straightforward due to the energy overlap between orbitals, especially as we move to higher energy levels.
For example, the 4s orbital is filled before the 3d orbital because it's at a slightly lower energy level. Understanding this principle is crucial for predicting and interpreting electron configurations.
The Valence Shell: The Seat of Chemical Activity
The valence shell is the outermost electron shell of an atom. It is the most important shell in determining an element's chemical properties.
For bromine, the valence shell is the fourth shell (n=4), containing the 4s and 4p subshells. This shell houses a total of seven valence electrons.
The number of valence electrons dictates how bromine will interact with other atoms to form chemical bonds.
The drive to achieve a stable electron configuration, usually resembling that of a noble gas, is what propels chemical reactions.
Significance in Chemical Bonding
The valence shell is the primary site of chemical bonding. Atoms interact with each other to achieve a stable electron configuration.
Bromine, with its seven valence electrons, is highly reactive because it is one electron short of achieving a full octet, a stable configuration with eight electrons in its valence shell.
This deficiency makes it eager to either gain an electron through ionic bonding or share electrons through covalent bonding.
Valence Electrons: Dictating Reactivity
Valence electrons are the electrons located in the outermost shell of an atom, also known as the valence shell.
These electrons are responsible for the element's chemical reactivity, including the types of bonds it forms.
Bromine's seven valence electrons are the reason behind its high reactivity. Bromine seeks to complete its octet.
The Drive for an Octet
Bromine exhibits a strong tendency to gain one electron to achieve a stable octet configuration. This drive underlies many of its chemical reactions.
By gaining an electron, bromine forms a negative ion, Br⁻, also known as a bromide ion. This ion has a stable electron configuration similar to that of the noble gas krypton (Kr).
This tendency explains bromine's propensity to form ionic bonds with metals. It readily accepts electrons from metals to form stable ionic compounds.
Chemical Bonding and Interactions: Understanding Bromine's Behavior
Having explored the atomic structure and electronic configuration of bromine, it becomes crucial to understand how this element interacts with others to form molecules and compounds. This section delves into the intricacies of chemical bonding involving bromine, examining its adherence to the octet rule, its representation using Lewis dot structures, and its participation in ionic and covalent bonds.
The Octet Rule and Bromine's Quest for Stability
The octet rule, a cornerstone of chemical bonding theory, dictates that atoms tend to gain, lose, or share electrons to achieve a full outer shell of eight electrons, resembling the stable electron configuration of noble gases.
Bromine, possessing seven valence electrons, exhibits a strong proclivity to acquire one additional electron to complete its octet. This tendency underpins its high reactivity and its propensity to form stable chemical bonds.
While bromine largely adheres to the octet rule, deviations can occur under specific circumstances. These exceptions often involve the formation of expanded octets, where the central bromine atom accommodates more than eight electrons in its valence shell. However, such instances are relatively rare and typically involve bonding with highly electronegative elements.
Lewis Dot Structures: Visualizing Bromine's Bonding
Lewis dot structures provide a visual representation of valence electrons and their involvement in chemical bonding.
For a neutral bromine atom (Br), the Lewis dot structure depicts the element symbol surrounded by seven dots, each representing a valence electron.
In the case of a diatomic bromine molecule (Br₂), two bromine atoms share a pair of electrons to form a single covalent bond, each achieving a complete octet. Similarly, in hydrogen bromide (HBr), a hydrogen atom shares an electron with a bromine atom, resulting in a single covalent bond and stable electron configurations for both atoms.
Bromine's Versatility in Chemical Bonding
Bromine exhibits remarkable versatility in forming diverse chemical bonds, engaging in both ionic and covalent interactions depending on the electronegativity of the interacting atoms.
Ionic Bonding: Formation of Bromides
When reacting with highly electropositive elements, such as alkali metals (e.g., sodium, potassium), bromine readily accepts an electron to form a negatively charged bromide ion (Br-).
This process results in the formation of ionic compounds, such as sodium bromide (NaBr) and potassium bromide (KBr), characterized by strong electrostatic attractions between oppositely charged ions.
Covalent Bonding: Sharing Electrons
Bromine also readily forms covalent bonds by sharing electrons with other nonmetals.
For instance, bromine reacts with hydrogen to form hydrogen bromide (HBr), a polar covalent compound where electrons are shared unequally due to the difference in electronegativity between hydrogen and bromine.
Bromine typically forms single covalent bonds, but in certain complex molecules, it can also participate in double bonds. However, triple bonds involving bromine are exceptionally rare due to the energy requirements and steric constraints involved.
Chemical Properties and Reactivity: Exploring Bromine's Reactive Nature
Having explored the atomic structure and electronic configuration of bromine, it becomes crucial to understand how this element interacts with others to form molecules and compounds. This section delves into the intricacies of chemical bonding involving bromine, examining its adherence to periodic trends and its proclivity for certain chemical reactions. The aim is to elucidate bromine's inherent reactivity and its consequential role in various chemical processes.
The Allure of the Bromide Ion (Br-)
Central to understanding bromine's reactivity is its propensity to form the bromide ion (Br-). This transformation occurs when a neutral bromine atom gains an electron, thereby achieving a stable octet configuration in its valence shell.
The process is energetically favorable due to bromine's high electronegativity, which dictates its strong attraction for electrons.
The resulting bromide ion carries a negative charge, rendering it an anion poised for ionic interactions.
Properties of Bromide Ions
Bromide ions exhibit several key characteristics. Their negative charge allows them to readily form ionic bonds with positively charged cations.
This interaction leads to the formation of stable ionic compounds such as sodium bromide (NaBr) and potassium bromide (KBr).
Furthermore, the stability of the bromide ion stems from its complete octet, making it relatively unreactive in isolation.
The Role of Bromide in Ionic Compounds
Bromide ions are integral components of numerous ionic compounds. These compounds often exhibit high melting points and conduct electricity when dissolved in water, owing to the presence of mobile ions.
In biological systems, bromide ions, albeit in small concentrations, can influence neuronal activity and play a role in certain enzymatic processes.
Periodic Trends: Decoding Bromine's Behavior
The periodic table provides a valuable framework for understanding bromine's chemical behavior. Its position as a halogen in Group 17 elucidates its high electronegativity and its tendency to gain electrons.
Electronegativity and Reactivity
Electronegativity, the measure of an atom's ability to attract electrons in a chemical bond, increases across the periodic table from left to right and decreases down a group.
Bromine's high electronegativity, relative to elements to its left, explains its readiness to accept electrons and form negative ions.
This strong attraction for electrons contributes to its high reactivity with metals and other electron-donating species.
Ionization Energy and Ion Formation
Ionization energy, the energy required to remove an electron from an atom, offers another lens through which to view bromine's behavior.
Bromine possesses a relatively high ionization energy, signifying that it requires a considerable amount of energy to lose an electron.
However, its electron affinity (the energy released when an electron is added) is also high, indicating that it readily accepts electrons.
This explains why bromine is more prone to forming anions rather than cations.
Bromine Compared to Other Halogens
Compared to its halogen brethren, chlorine (Cl) and iodine (I), bromine occupies an intermediate position in terms of reactivity.
Chlorine is generally more reactive than bromine due to its higher electronegativity and smaller atomic size. Conversely, iodine is less reactive than bromine due to its lower electronegativity and larger atomic size.
Unveiling Bromine's Reactions
Bromine engages in a diverse array of chemical reactions, driven by its electron-seeking nature.
Reactions with Metals: Forming Metal Bromides
Bromine reacts vigorously with many metals, forming metal bromides. For example, the reaction between bromine and sodium yields sodium bromide (NaBr), an ionic compound with a crystalline structure.
These reactions are typically exothermic, releasing heat and often producing vibrant displays of light.
Reactions with Hydrocarbons: The Halogenation Process
Bromine also reacts with hydrocarbons, particularly unsaturated ones (containing double or triple bonds), through a process called halogenation.
This reaction involves the addition of bromine atoms to the carbon-carbon multiple bond, resulting in a saturated compound.
Halogenation reactions are crucial in organic chemistry for synthesizing various brominated compounds.
Noteworthy Reactions
While the above reactions exemplify bromine's typical behavior, certain unique reactions are worth noting. Bromine can act as an oxidizing agent in some reactions, accepting electrons from other species and causing them to be oxidized.
Its reactions with ammonia and certain organic compounds can yield explosive products under specific conditions, highlighting the importance of handling bromine with care.
Applications of Bromine: Bromine's Real-World Impact
Having established a foundational understanding of bromine's properties and reactivity, it's time to explore the tangible ways this element shapes our world. Bromine and its compounds manifest in remarkably diverse applications, spanning industries from fire safety to pharmaceuticals and agriculture. This section seeks to illuminate the practical significance of bromine, showcasing its benefits, and also acknowledging its environmental challenges.
Flame Retardants: A Critical Component of Fire Safety
Bromine's most significant application lies in its role as a flame retardant. Brominated flame retardants (BFRs) are incorporated into a wide array of materials to inhibit or suppress combustion.
These compounds function by interfering with the chemical processes that sustain a fire. When a material containing a BFR is exposed to heat, the BFR releases bromine radicals.
These radicals scavenge highly reactive hydrogen and hydroxyl radicals in the gas phase, effectively slowing down or stopping the chain reaction of combustion.
Ubiquitous Use in Everyday Products
BFRs are extensively used in plastics, textiles, and electronics.
They are commonly found in:
- Electronic equipment casings (computers, televisions).
- Upholstery and textiles used in furniture.
- Building materials and insulation.
- Automotive components.
This widespread use aims to reduce the flammability of these materials, offering crucial protection against fire hazards and potentially saving lives.
Environmental Considerations and Regulatory Scrutiny
While BFRs have undoubtedly contributed to enhanced fire safety, their environmental impact has come under increasing scrutiny.
Certain BFRs have been shown to be persistent in the environment, bioaccumulative in living organisms, and potentially toxic.
This has led to regulatory actions, including bans or restrictions on the use of specific BFRs in various regions. Ongoing research focuses on developing safer and more environmentally friendly alternatives to traditional BFRs.
Pharmaceuticals: Bromine's Role in Drug Development
Bromine plays a surprisingly important role in the pharmaceutical industry. The incorporation of bromine atoms into drug molecules can significantly alter their properties.
Bromine can:
- Increase the drug's lipophilicity (fat solubility), affecting its absorption and distribution in the body.
- Enhance the drug's binding affinity to its target protein, leading to increased efficacy.
- Alter the drug's metabolic stability, potentially prolonging its duration of action.
Notable Examples of Bromine-Containing Pharmaceuticals
Several commercially available drugs contain bromine, demonstrating its value in drug development:
- Verapamil: An antianginal and antihypertensive drug.
- Bromfenac: A nonsteroidal anti-inflammatory drug (NSAID).
- Halothane: An older general anesthetic (though its use has declined due to the availability of safer alternatives).
The presence of bromine in these drugs is not merely incidental. In many cases, it contributes directly to their therapeutic efficacy and pharmacological profile.
Agriculture: Balancing Crop Protection with Environmental Responsibility
In agriculture, bromine has historically been used as a soil fumigant to control pests and diseases. Methyl bromide, in particular, was widely used to sterilize soil prior to planting, eliminating a broad spectrum of nematodes, fungi, insects, and weeds.
The Phase-Out of Methyl Bromide
However, methyl bromide is a potent ozone-depleting substance. Under the Montreal Protocol, a global agreement to protect the ozone layer, methyl bromide has been phased out in most countries.
Current Regulations and Alternatives
The use of methyl bromide is now severely restricted, with limited exemptions for specific critical uses where no viable alternatives exist. Research and development efforts are focused on finding safer and more sustainable alternatives to methyl bromide for soil fumigation.
These alternatives include:
- Other chemical fumigants (with lower ozone-depletion potential).
- Biological control agents.
- Integrated pest management strategies.
Other Applications: Disinfectants and Industrial Processes
Beyond flame retardants, pharmaceuticals, and agriculture, bromine finds use in a variety of other applications:
Disinfection
Bromine compounds, such as bromochlorodimethylhydantoin (BCDMH), are used as disinfectants in swimming pools and spas.
Bromine offers several advantages over chlorine as a disinfectant:
- It is more effective at higher pH levels.
- It produces fewer irritating byproducts.
- It has a longer active life.
Industrial Chemistry
Bromine compounds serve as important reactants and intermediates in various industrial processes. They are used in the synthesis of dyes, photographic chemicals, and a range of organic compounds. The unique reactivity of bromine makes it a valuable tool in chemical synthesis.
Video: Bromine Valence Electrons: A Student's Guide
FAQs: Bromine Valence Electrons
What are valence electrons, and why are they important for bromine?
Valence electrons are the electrons in the outermost shell of an atom. They are important for bromine because they determine how bromine interacts with other atoms to form chemical bonds. The number of bromine valence electrons dictates its reactivity.
How many valence electrons does bromine have?
Bromine has 7 valence electrons. This means it needs only one more electron to achieve a stable octet configuration.
Why does bromine tend to form single bonds?
Bromine tends to form single bonds because it has 7 valence electrons and readily accepts one more electron to achieve a stable electron configuration. By sharing one electron with another atom, bromine valence electrons complete its outer shell.
Where can I find bromine on the periodic table, and how does its location relate to its valence electrons?
Bromine is located in Group 17 (also known as the Halogens) on the periodic table. Elements in the same group have the same number of valence electrons. All halogens, including bromine, have 7 valence electrons.
So, there you have it! Hopefully, this guide has made understanding bromine valence electrons a little less daunting. Now you can confidently tackle those chemistry problems and impress your teachers (or at least not feel totally lost!). Good luck!