Bromine NMR: Principles, Apps & Interpretation
Bromine NMR spectroscopy, a technique greatly enhanced by advancements in instrumentation such as the Bruker Avance III HD, provides unique insights into molecular structures and dynamics that complement traditional proton and carbon NMR. The inherent quadrupolar nature of bromine nuclei, particularly isotopes like bromine-79, often leads to broadened signals, necessitating sophisticated acquisition and processing methods to extract meaningful data. Researchers at institutions like the University of Oxford have been instrumental in developing novel techniques to overcome these challenges, expanding the applicability of bromine NMR in fields ranging from polymer chemistry to pharmaceutical research. Understanding the principles of relaxation mechanisms is crucial for accurate spectral interpretation, allowing for the determination of parameters such as correlation times and quadrupolar coupling constants, thereby unlocking the full potential of bromine nmr in structural elucidation.
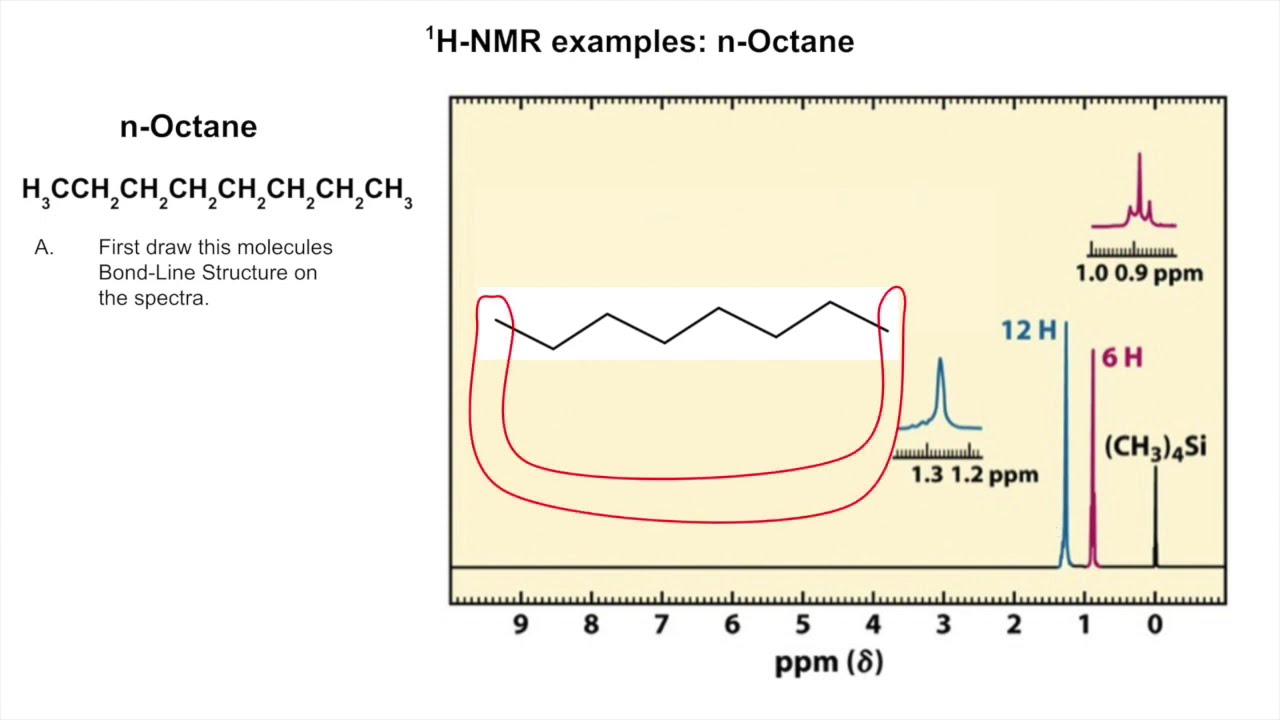
Image taken from the YouTube channel Mark Springsteel , from the video titled H-NMR Example of n-Octane and Bromocyclohexane .
Nuclear Magnetic Resonance (NMR) spectroscopy stands as a cornerstone analytical technique in various scientific disciplines, offering unparalleled insights into molecular structure, dynamics, and interactions. This section serves as an introduction to the specific realm of Bromine NMR, highlighting its unique characteristics and applications.
The Foundation: Nuclear Magnetic Resonance (NMR)
At its core, NMR exploits the magnetic properties of atomic nuclei. Nuclei with an odd number of protons or neutrons possess an intrinsic angular momentum, or spin, which generates a magnetic moment.
When placed in a strong external magnetic field, these nuclei align either with or against the field. Applying radiofrequency radiation can induce transitions between these energy levels.
The frequencies at which these transitions occur are highly sensitive to the local electronic environment of the nucleus, providing a wealth of information about molecular structure and dynamics.
NMR's Pervasive Influence
NMR's versatility has cemented its importance across numerous fields. In chemistry, it is indispensable for elucidating the structure of newly synthesized compounds and understanding reaction mechanisms.
Biochemistry relies heavily on NMR to study the structure and dynamics of proteins, nucleic acids, and other biomolecules. Materials science leverages NMR to characterize polymers, ceramics, and other materials, probing their composition and properties.
The non-destructive nature and high information content of NMR make it an invaluable tool for both fundamental research and industrial applications.
Diving Deeper: Bromine NMR
While proton and carbon NMR are widely utilized, Bromine NMR presents a unique set of challenges and opportunities. Bromine has two NMR-active isotopes: bromine-79 (⁷⁹Br) and bromine-81 (⁸¹Br).
These isotopes share similar NMR properties, but differ slightly in their resonance frequencies and natural abundance. Both are quadrupolar nuclei.
This quadrupolar nature fundamentally influences the appearance and interpretation of bromine NMR spectra.
The Allure and Obstacles of Bromine NMR
Compared to proton or carbon NMR, bromine NMR offers some distinct advantages. The sensitivity of bromine chemical shifts to subtle changes in the electronic environment can provide valuable information about molecular structure and bonding.
Moreover, the presence of bromine in a molecule can serve as a strategically placed "reporter group," offering a unique vantage point for probing specific regions of interest.
However, the quadrupolar nature of bromine also introduces significant challenges. Quadrupolar relaxation is the dominant relaxation mechanism for bromine nuclei.
This leads to rapid signal decay and consequently, significantly broadened spectral lines. This broadening can reduce spectral resolution and sensitivity, making it more challenging to resolve distinct signals and obtain quantitative data.
Despite these challenges, bromine NMR remains a powerful technique when applied judiciously. Its unique sensitivity to the bromine environment, combined with carefully optimized experimental parameters, allows researchers to gain valuable insights into molecular structure, dynamics, and reactivity.
Theoretical Underpinnings: Understanding Bromine NMR Spectra
Nuclear Magnetic Resonance (NMR) spectroscopy stands as a cornerstone analytical technique in various scientific disciplines, offering unparalleled insights into molecular structure, dynamics, and interactions. This section serves as an introduction to the specific realm of Bromine NMR, highlighting its unique characteristics and applications.
The interpretation of Bromine NMR spectra requires a solid grasp of the theoretical principles governing the behavior of bromine nuclei under the influence of a magnetic field.
Bromine, with its quadrupolar isotopes, presents both challenges and opportunities compared to more commonly studied nuclei like protons or carbon-13. Understanding these differences is crucial for effective data acquisition and analysis.
Quadrupole Nuclei and Quadrupolar Relaxation
Bromine possesses two NMR-active isotopes, 79Br and 81Br, both of which are quadrupolar nuclei.
This means that their nuclear spin quantum number (I) is greater than 1/2. Specifically, both have I = 3/2. Unlike nuclei with a spherical charge distribution, quadrupolar nuclei possess an ellipsoidal charge distribution.
This asymmetry leads to a nuclear quadrupole moment, which interacts strongly with electric field gradients (EFGs) present in the molecule. These EFGs arise from the surrounding electron distribution and bonding environment.
This interaction is the basis for quadrupolar relaxation, the dominant relaxation mechanism for bromine nuclei.
Quadrupolar relaxation arises from the fluctuating electric field gradients at the nucleus due to molecular motions and vibrations. These fluctuations induce transitions between the nuclear spin states, causing rapid relaxation back to equilibrium.
The Mechanism of Quadrupolar Relaxation
The efficiency of quadrupolar relaxation depends on the magnitude of the quadrupole moment and the strength and correlation time of the EFG. Smaller, symmetrical molecules tend to have longer relaxation times, while larger, asymmetric molecules have shorter relaxation times.
Consequences of Quadrupolar Relaxation: Line Broadening
The rapid quadrupolar relaxation has a profound impact on the appearance of bromine NMR spectra. Specifically, it leads to significant line broadening.
According to the Heisenberg uncertainty principle, the shorter the lifetime of a spin state, the broader its corresponding spectral line.
Since quadrupolar relaxation shortens the lifetime of the bromine nuclear spin states, the resulting NMR signals are often broadened, sometimes to the point where they become difficult to detect.
Impact on Resolution and Sensitivity
Line broadening dramatically affects both spectral resolution and sensitivity. Overlapping signals may become indistinguishable, hindering the accurate determination of chemical shifts and coupling constants.
Furthermore, broad lines reduce the signal-to-noise ratio, making it more challenging to detect bromine resonances, especially at low concentrations. This is one of the main difficulties in utilizing bromine NMR.
Chemical Shift in Bromine NMR
Despite the line broadening challenges, chemical shifts in bromine NMR provide valuable information about the electronic environment surrounding the bromine atom.
The chemical shift is sensitive to factors such as electronegativity of neighboring atoms, bond order, and the presence of lone pairs.
Electronegative substituents deshield the bromine nucleus, resulting in a downfield shift (higher ppm value). Conversely, electron-donating groups shield the bromine nucleus, leading to an upfield shift (lower ppm value).
Referencing Standards
Accurate chemical shift referencing is crucial for comparing spectra and interpreting trends. Typically, bromine NMR spectra are referenced to external standards.
Commonly used standards include solutions of KBr or other simple bromine salts. The choice of standard and solvent should be carefully considered to minimize variations due to solvent effects and concentration dependence.
Relaxation Times (T1, T2)
As previously mentioned, relaxation times play a pivotal role in bromine NMR. The longitudinal relaxation time (T1) describes the time it takes for the nuclear spin magnetization to return to its equilibrium value along the direction of the magnetic field.
The transverse relaxation time (T2) characterizes the decay of the nuclear spin magnetization in the plane perpendicular to the magnetic field.
In bromine NMR, T2 is typically much shorter than T1 due to the dominance of quadrupolar relaxation. This difference contributes to the observed line broadening.
Measuring Relaxation Times
Measuring T1 and T2 relaxation times can provide insights into the dynamics and interactions of bromine-containing molecules.
Inversion recovery experiments are commonly used to determine T1 values, while spin-echo experiments (Carr-Purcell-Meiboom-Gill sequence) are used to measure T2 values.
Solvent Effects and Temperature Dependence
Solvent properties and temperature can significantly influence bromine NMR parameters. Solvent polarity, viscosity, and the presence of specific interactions between the solvent and the bromine-containing molecule can all affect chemical shifts and relaxation rates.
Higher temperatures generally lead to faster molecular motions, which can affect quadrupolar relaxation and linewidths. Careful temperature control is essential for obtaining reproducible and reliable bromine NMR data.
Experimental Design Considerations
When designing bromine NMR experiments, it is important to consider these solvent and temperature effects.
Choosing an appropriate solvent that minimizes interactions with the analyte and maintaining a stable temperature are crucial for obtaining high-quality spectra.
Linewidth Analysis
Despite the challenges posed by line broadening, linewidth analysis can provide valuable information about molecular dynamics and the chemical environment surrounding the bromine atom.
By carefully measuring and analyzing the linewidths of bromine NMR signals, it is possible to gain insights into the rates of molecular motions, the strength of intermolecular interactions, and the degree of homogeneity in the sample.
Mathematical Models and Interpretation
Several mathematical models can be used to interpret linewidth data. These models relate the linewidth to factors such as the quadrupolar coupling constant, the correlation time for molecular motions, and the viscosity of the solution.
Careful interpretation of these models can provide a deeper understanding of the structure and dynamics of bromine-containing molecules.
Experimental Considerations: Setting Up for Success
Having established a theoretical understanding of Bromine NMR, it is crucial to translate this knowledge into practical experimental design. The following section details the key considerations for successful execution of Bromine NMR experiments, covering aspects from instrumentation and sample preparation to pulse sequence optimization and referencing.
NMR Spectrometers and NMR Tubes
Achieving high-quality Bromine NMR spectra demands careful attention to spectrometer configuration and sample handling.
The ideal spectrometer setup for Bromine NMR should be equipped with a broadband probe capable of tuning to the resonance frequency of the bromine isotope of interest, typically either 79Br or 81Br.
Cryoprobes can significantly enhance sensitivity due to reduced thermal noise.
Ensure the probe is properly matched and tuned to the bromine frequency for optimal signal transmission and reception.
When choosing NMR tubes, select high-quality tubes with consistent wall thickness to minimize distortions in the magnetic field.
Sample preparation is paramount for obtaining reliable results.
The solute should be dissolved in a suitable deuterated solvent that does not interfere with the bromine signal and provides adequate solubility.
The concentration of the bromine-containing compound should be optimized to balance signal intensity and spectral resolution.
Avoid high concentrations that can lead to increased viscosity and line broadening.
Additionally, ensure that the sample is free from paramagnetic impurities, which can drastically reduce signal quality.
Thoroughly degas the sample to remove dissolved oxygen, as oxygen is paramagnetic and can cause signal broadening.
Pulse Sequences
Optimizing pulse sequences is vital to maximize signal-to-noise ratio and minimize the effects of rapid quadrupolar relaxation.
A simple single pulse experiment is often sufficient for acquiring basic 1D Bromine NMR spectra.
However, due to the short relaxation times associated with quadrupolar nuclei, careful adjustment of pulse width and recycle delay is necessary.
Shorter pulse widths are generally preferred to excite a broader range of frequencies and compensate for rapid signal decay.
The recycle delay should be optimized to allow for complete relaxation of the bromine nuclei between scans, ensuring accurate signal integration.
Several advanced pulse sequences can be tailored for quadrupolar nuclei to improve spectral resolution and sensitivity.
One commonly used technique is the quadrupolar echo sequence, which can refocus broadened signals and improve the signal-to-noise ratio.
Other techniques, such as inverse detected experiments, can be employed to indirectly detect bromine signals through more sensitive nuclei, such as protons or carbons.
Shimming
Achieving optimal magnetic field homogeneity is crucial for obtaining high-resolution Bromine NMR spectra.
Inhomogeneous magnetic fields can lead to significant line broadening, which is particularly detrimental for quadrupolar nuclei with already broad spectral lines.
Therefore, careful shimming of the magnet is essential to minimize these effects.
Manual or automatic shimming routines can be employed to optimize the homogeneity of the magnetic field.
Pay particular attention to shims that correct for field gradients in the z-direction, as these typically have the most significant impact on spectral resolution.
It may be necessary to adjust shims iteratively to achieve the best possible lineshape.
Standard Reference Compounds
Accurate chemical shift referencing is necessary for comparing Bromine NMR data across different experiments and laboratories.
The choice of appropriate reference compounds is critical for ensuring the reliability of chemical shift assignments.
External referencing is a common approach in Bromine NMR, where a sealed capillary containing a known bromine-containing compound is inserted into the NMR tube.
Suitable reference compounds include solutions of KBr or NaBr in D2O.
The chemical shift of the reference compound is then used to calibrate the entire spectrum.
When using external referencing, ensure that the reference compound is properly sealed and that the capillary is positioned consistently within the NMR tube.
Alternatively, internal referencing can be employed if a suitable reference compound is present within the sample itself.
In this case, the chemical shift of the reference compound is directly measured from the same spectrum as the analyte.
Regardless of the referencing method, it is essential to clearly document the reference compound used and its chemical shift value to ensure reproducibility and comparability of results.
Applications of Bromine NMR: Real-World Examples
Having explored the nuances of experimental considerations, it's time to illustrate the utility of Bromine NMR through real-world applications. This section showcases the diverse applications of bromine NMR across various fields, highlighting its utility in understanding molecular structure, dynamics, and interactions.
Bromine NMR in Chemical Compound Analysis
Bromine NMR is applicable to diverse chemical compounds, offering unique insights into their structure and behavior.
Elemental Bromine (Br2)
While perhaps less common, NMR of elemental bromine can provide information on its electronic environment and interactions with solvents. The extreme reactivity and corrosive nature of Br2 necessitate careful handling and specialized experimental setups.
Inorganic Bromides
Inorganic bromides, such as sodium bromide (NaBr) and potassium bromide (KBr), are widely used in various applications. Bromine NMR can be employed to study their solvation dynamics and interactions with other ions in solution. The linewidth of the bromine signal provides information on the symmetry of the bromide ion's environment and its rate of exchange with the bulk solvent.
Organobromides
Organobromides are prevalent in organic synthesis and are widely used as building blocks for more complex molecules. Bromine NMR is particularly valuable for characterizing these compounds, providing information on the electronic environment around the bromine atom and the stereochemistry of the molecule.
Variations in chemical shifts can distinguish between different types of organobromides (e.g., alkyl, aryl, vinyl bromides).
Brominated Polymers
Brominated polymers are commonly used as flame retardants in plastics and textiles. Bromine NMR is a powerful tool for characterizing these materials, providing information on the degree of bromination, the distribution of bromine atoms within the polymer chain, and the thermal stability of the brominated polymer.
Bromine-Containing Pharmaceuticals
Many pharmaceuticals contain bromine atoms, which can influence their biological activity and pharmacokinetic properties. Bromine NMR can be used to study the structure and dynamics of these drug molecules, as well as their interactions with biological targets.
Probing Coordination Chemistry with Bromine NMR
Bromine NMR plays a vital role in understanding the intricate world of coordination chemistry.
It provides valuable insights into metal-bromine interactions and the structures of complex compounds.
Understanding Metal-Bromine Interactions
Bromine NMR can reveal important details about the bonding and electronic environment in metal-bromine complexes. Changes in the bromine chemical shift and linewidth can indicate the strength of the metal-bromine bond and the coordination geometry around the metal center.
Catalysis and Materials Science Applications
The information gleaned from bromine NMR studies of coordination complexes finds direct application in catalysis and materials science. For instance, understanding the interaction between a metal catalyst and a brominated substrate can help optimize catalytic reactions.
In materials science, tailoring the metal-bromine interaction can influence the properties of new materials.
Brominated Materials in Materials Science
Bromine NMR has a significant role in the characterization of brominated polymers and flame retardants, crucial for material safety and performance.
Characterization of Brominated Polymers
Bromine NMR offers specific data on the degree and pattern of bromination in polymers.
It helps determine how effectively bromine is incorporated into the polymer structure, which is crucial for their functionality.
Investigating Material Properties
This technique is valuable for researching relationships between bromination and the properties of materials. By observing the bromine environment, researchers can tune polymers for optimal flame retardancy and durability.
Pharmaceutical Insights Through Bromine NMR
Bromine-containing pharmaceuticals benefit greatly from the analytical power of bromine NMR.
Analyzing Drug Molecules and Interactions
Bromine NMR allows scientists to analyze the structure and behavior of bromine-containing drugs.
It offers insights into how these molecules interact with their targets and their behavior in biological systems.
Applications in Drug Discovery and Development
In drug discovery, Bromine NMR aids in developing safer and more effective pharmaceuticals. Understanding bromine's effects within a molecule can lead to improved drug designs and applications.
Determining Solution Structure using Bromine NMR Data
Solution Structure of Molecules
Bromine NMR aids in determining the precise structure of molecules within solutions, giving a clear picture of molecular arrangement and behavior.
Combining NMR and Computational Methods
Using NMR data alongside computational techniques greatly enhances structural analysis. These combined efforts produce robust models that show detailed molecular interactions, providing a thorough understanding of bromine-containing compounds in action.
Applications of Bromine NMR: Real-World Examples
Having explored the nuances of experimental considerations, it's time to illustrate the utility of Bromine NMR through real-world applications. This section showcases the diverse applications of bromine NMR across various fields, highlighting its utility in understanding molecular structure, dynamics, and interactions.
Data Processing and Analysis: Extracting Meaning from Spectra
The true power of any spectroscopic technique lies not only in its ability to generate data but also in the meticulous processing and insightful analysis of that data.
For Bromine NMR, this is especially crucial given the inherent challenges associated with quadrupolar relaxation and the resulting broad spectral lines. This section outlines the essential steps involved in processing and analyzing bromine NMR data, ensuring accurate and reliable interpretations.
Data Processing Software
The initial step in extracting meaningful information from Bromine NMR spectra involves the use of specialized software designed for NMR data processing. Several robust and widely used software packages are available, each offering a suite of tools tailored to address the specific challenges of NMR data analysis.
These packages include:
-
MestReNova (Mnova): Known for its user-friendly interface and comprehensive processing capabilities.
-
TopSpin: A powerful platform with advanced features for spectral analysis.
-
NMRPipe: Favored for its flexibility and scripting capabilities, often used in research settings.
-
Chenomx NMR Suite: A comprehensive package particularly useful for metabolomics and quantitative NMR.
Essential Processing Techniques
Within these software environments, several key techniques are applied to transform the raw data into a usable and interpretable format.
Baseline Correction: A fundamental step to remove unwanted background signals and distortions, ensuring accurate peak integration and analysis. This is crucial in Bromine NMR due to the broad signals.
Phasing: Adjusting the spectrum to ensure that all signals are displayed in their correct, absorptive mode. Accurate phasing is critical for proper integration and chemical shift determination.
Peak Fitting: Employing mathematical models to deconvolute overlapping peaks and extract precise parameters such as chemical shift, linewidth, and integral. Given the broadened lines in bromine NMR, sophisticated peak fitting algorithms are indispensable.
Best Practices for NMR Data Acquisition and Processing
To ensure that the subsequent data processing and analysis yield reliable and reproducible results, adhering to best practices during data acquisition and processing is paramount.
Guidelines for Reliable Results
Several guidelines should be followed to guarantee high-quality data:
-
Careful Sample Preparation: Ensuring the sample is properly dissolved and free from contaminants is essential. Inconsistent sample preparation can lead to artifacts in the data.
-
Appropriate Spectrometer Settings: Optimizing parameters such as pulse width, acquisition time, and spectral width is crucial. These settings directly impact the signal-to-noise ratio and spectral resolution.
-
Sufficient Signal Averaging: Acquiring enough scans to achieve an adequate signal-to-noise ratio is vital, especially for bromine NMR due to its inherent sensitivity limitations.
-
Proper Shimming: Achieving and maintaining magnetic field homogeneity (shimming) is fundamental to obtaining well-resolved spectra.
Error Analysis and Quality Control
Even with careful execution, errors can arise during data acquisition and processing. Rigorous error analysis and quality control measures are necessary to validate the data.
-
Signal-to-Noise Ratio (SNR) Assessment: Evaluating the SNR provides an indication of the data's quality and reliability. Low SNR can compromise the accuracy of peak identification and quantification.
-
Linewidth Consistency: Monitoring the linewidth of reference peaks throughout the data set can reveal inconsistencies or instrumental issues.
-
Residual Analysis: Examining the residuals after peak fitting can uncover systematic errors or inadequacies in the fitting model.
-
Reproducibility Checks: Comparing spectra acquired under identical conditions can verify the reproducibility of the experiment and identify potential sources of error.
By diligently implementing these data processing and analysis techniques, researchers can unlock the rich information encoded within Bromine NMR spectra, gaining valuable insights into molecular structure, dynamics, and interactions.
Video: Bromine NMR: Principles, Apps & Interpretation
Frequently Asked Questions about Bromine NMR
Why is bromine NMR less common than proton or carbon NMR?
Bromine has a quadrupolar nucleus, leading to rapid relaxation. This results in broad signals in bromine nmr spectra, which can be difficult to resolve and interpret. Consequently, other techniques are often preferred.
What information can bromine NMR provide?
Bromine nmr can determine the presence and electronic environment of bromine atoms in a molecule. It's useful for studying brominated compounds and reaction mechanisms involving bromine, despite the challenges posed by signal broadening.
What are the major challenges in acquiring and interpreting bromine NMR spectra?
The primary challenges stem from the quadrupolar nature of bromine isotopes, which causes rapid relaxation and broad signals. This necessitates specialized techniques, longer acquisition times, and careful consideration during spectral interpretation of bromine nmr data.
What are some specific applications of bromine NMR?
Bromine nmr finds application in characterizing brominated polymers, investigating the binding of bromide ions to proteins, and studying reaction intermediates in bromination reactions. Although less routine than other NMR techniques, it provides valuable insight when dealing with bromine-containing compounds.
So, next time you're facing a structural conundrum and good ol' proton NMR just isn't cutting it, remember the power of bromine NMR! It might not be the first technique that springs to mind, but with a little know-how, it can be a real game-changer for unlocking the secrets hidden within your molecules. Happy analyzing!