Boc Deprotection: The Expert's Complete How-To Guide
tert-Butyloxycarbonyl (Boc) deprotection, a crucial reaction in peptide synthesis, facilitates the removal of the Boc protecting group under acidic conditions. Trifluoroacetic acid (TFA), a common reagent, is frequently employed for selective cleavage of the Boc group from amino acids and peptides. Bachem, a prominent supplier of peptides and biochemicals, offers a wide range of Boc-protected amino acids essential for chemical synthesis. Understanding the nuances of boc deprotection is critical for researchers performing reactions in laboratories focused on peptide chemistry.
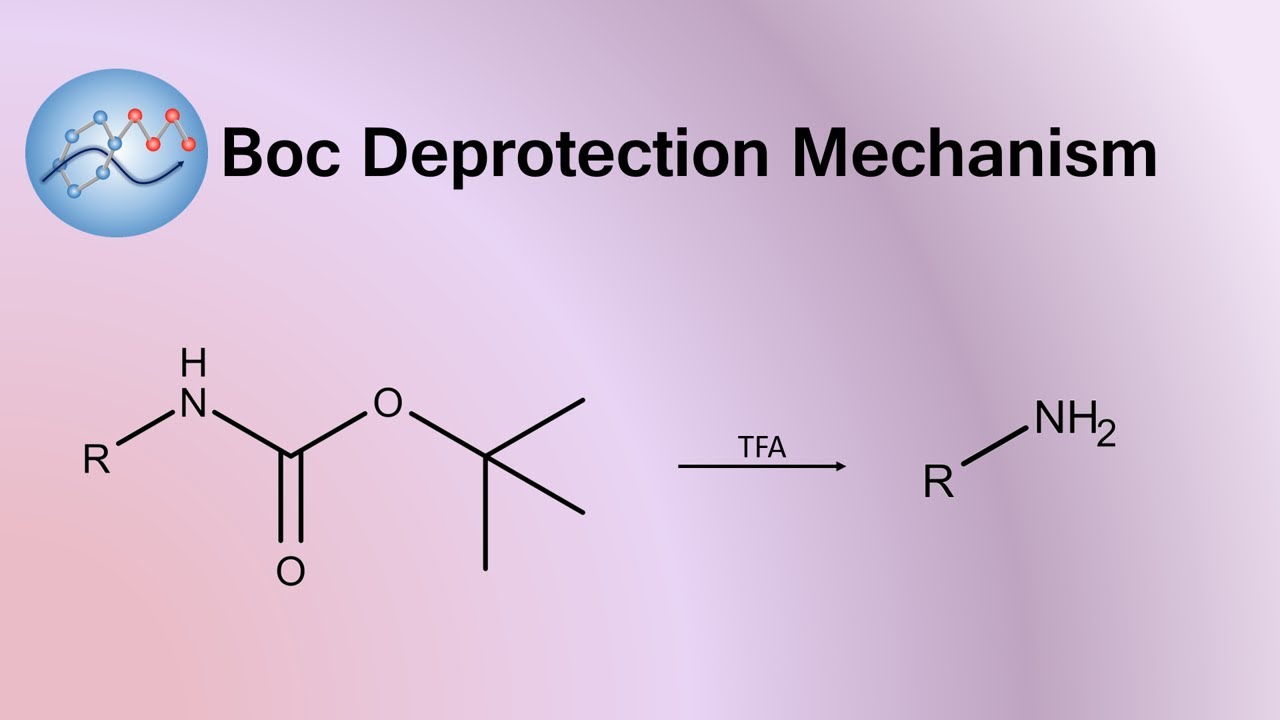
Image taken from the YouTube channel Organic Mechanisms , from the video titled Boc Deprotection Mechanism | Organic Chemistry .
The tert-Butyloxycarbonyl (Boc) protecting group stands as a cornerstone in modern organic synthesis, particularly in the realm of peptide chemistry. Its strategic application allows chemists to selectively block amine functionalities, enabling controlled reactions at other sites within a molecule.
The subsequent removal of this protecting group, a process known as Boc deprotection, is not merely a final step, but a pivotal transformation that dictates the success and outcome of the entire synthetic sequence. Understanding the nuances of Boc deprotection is therefore essential for any chemist venturing into the synthesis of complex molecules.
What is the Boc Protecting Group?
The Boc group is a carbamate derivative characterized by a tert-butyl ester linked to a carbonyl group. Its chemical formula is (CH3)3COCO-. This bulky, electron-rich group is typically introduced onto amines via reaction with Boc anhydride ((Boc)2O) or Boc-ON (2-(tert-Butoxycarbonyloxyimino)-2-phenylacetonitrile).
The effectiveness of the Boc group stems from its unique chemical properties. It is remarkably stable under a wide range of reaction conditions, including those involving bases, nucleophiles, and metal catalysts.
However, it is readily cleaved under acidic conditions, a feature that allows for its selective removal without affecting other acid-labile protecting groups that may be present.
Why is Boc Used? Advantages in Organic and Peptide Synthesis
The widespread adoption of the Boc group in organic and peptide synthesis is attributable to several key advantages:
-
Orthogonality: Boc can be selectively removed in the presence of other protecting groups that are stable to acidic conditions, such as the benzyloxycarbonyl (Cbz) group or benzyl ethers. This orthogonality is crucial for complex multi-step syntheses.
-
Ease of Introduction: The reagents required for Boc protection, such as Boc anhydride and Boc-ON, are commercially available and relatively easy to handle. The reactions typically proceed in high yield under mild conditions.
-
Stability: The Boc group provides robust protection against a variety of reagents and reaction conditions commonly encountered in organic synthesis, thus minimizing undesired side reactions.
-
Peptide Synthesis Applicability: In peptide synthesis, the Boc group plays a critical role as an N-terminal protecting group, directing the sequential addition of amino acids to construct the desired peptide sequence. It's stability under the conditions used to couple amino acids and its ease of removal by acid without racemization makes it an ideal choice.
The Critical Role of Deprotection in Achieving Desired Product Purity and Yield
The deprotection step is where the protecting group is cleaved, revealing the amine functionality. This seemingly simple step is often fraught with challenges that can significantly impact the overall yield and purity of the final product.
Incomplete deprotection can lead to mixtures of protected and deprotected products, making purification difficult.
Furthermore, harsh deprotection conditions can lead to unwanted side reactions, such as peptide bond cleavage, degradation of sensitive functionalities, or the formation of byproducts that are difficult to remove.
Therefore, careful consideration must be given to the choice of deprotection reagent, reaction conditions, and workup procedures to ensure that the Boc group is removed cleanly and efficiently, thereby paving the way for a successful and high-yielding synthesis.
The effectiveness of the Boc group stems from its unique chemical properties. It is remarkably stable under a wide range of reaction conditions, including those involving bases, nucleophiles, and metal catalysts.
However, it is readily cleaved under acidic conditions, a feature that allows for its selective removal without affecting other acid-labile protecting groups that may be present.
With the Boc group strategically placed, and the desired reactions completed, the moment arrives to unveil the amine functionality once more. This is where the selection of the appropriate deprotection reagent becomes paramount, influencing the success and purity of the final product.
Choosing the Right Deprotection Reagent: A Comprehensive Guide
The removal of the Boc group, while seemingly straightforward, requires careful consideration of the available deprotection reagents. The choice is not arbitrary; it hinges on factors like substrate sensitivity, the presence of other functional groups, and the desired reaction conditions.
Overview of Deprotection Reagents: Key Considerations for Selection
Several reagents can accomplish Boc deprotection, but acids, particularly trifluoroacetic acid (TFA) and hydrochloric acid (HCl), are the most commonly employed. Lewis acids also find application in specific scenarios.
Selecting the optimal reagent necessitates evaluating several factors:
- Compatibility: The reagent must be compatible with the substrate and any other protecting groups present.
- Reaction Conditions: Temperature, solvent, and reaction time all play a crucial role.
- Ease of Use: Consider the handling, toxicity, and disposal of the reagent.
- Cost: The economic aspect can influence reagent selection, especially in large-scale syntheses.
Acids as Deprotection Reagents
Acids are the workhorses of Boc deprotection due to their effectiveness and relative ease of use. Both TFA and HCl are protic acids that protonate the carbamate oxygen, initiating the cleavage of the Boc group.
Trifluoroacetic Acid (TFA)
TFA is a strong organic acid widely used for Boc deprotection.
Mechanism: TFA protonates the carbamate oxygen of the Boc group, leading to the formation of tert-butyl cation and carbamic acid. The carbamic acid then decomposes to carbon dioxide and the free amine.
Conditions: TFA is typically used neat (undiluted) or in a solvent such as dichloromethane (DCM). Reactions are usually performed at room temperature or 0 °C.
Advantages:
- Rapid reaction rates.
- Effective deprotection in most cases.
- Soluble in many organic solvents.
Disadvantages:
- Highly corrosive and requires careful handling.
- Can cause side reactions, such as tert-butylation of aromatic rings or other nucleophilic sites.
- Difficult to remove completely due to its volatility and acidity.
- Can be incompatible with certain acid-sensitive functionalities.
Hydrochloric Acid (HCl)
HCl, a strong mineral acid, provides an alternative to TFA.
Mechanism: Similar to TFA, HCl protonates the carbamate oxygen, leading to Boc cleavage and the formation of the free amine.
Conditions: HCl is typically used as a solution in water, dioxane, or ethyl acetate. Reactions are often performed at room temperature or elevated temperatures.
Advantages:
- Relatively inexpensive.
- Effective for Boc deprotection.
- Can be easier to remove than TFA due to its higher boiling point and ionic nature.
Disadvantages:
- Can cause protonation of other basic sites in the molecule.
- May require longer reaction times than TFA.
- Incompatible with some acid-sensitive functionalities.
- The presence of water can lead to hydrolysis of other protecting groups or functional groups.
Lewis Acids
Lewis acids, such as boron trifluoride (BF3) or aluminum chloride (AlCl3), offer another avenue for Boc deprotection, although less commonly utilized than protic acids.
Mechanism: Lewis acids activate the carbonyl group of the Boc moiety by coordination, increasing its susceptibility to nucleophilic attack, such as from halide ions, leading to its eventual cleavage.
Conditions: These reactions typically require anhydrous conditions and inert solvents.
Advantages:
- Can be useful when protic acids are incompatible with the substrate.
- May offer higher selectivity in certain cases.
Disadvantages:
- Generally require anhydrous conditions, which can be challenging to maintain.
- Can be more difficult to handle than protic acids.
- May promote undesired side reactions, such as Friedel-Crafts alkylations.
- Often require careful optimization of reaction conditions.
When to Consider Lewis Acids:
Lewis acids are best considered when the substrate is highly sensitive to protic acids or when other protecting groups present are labile to protic conditions. They can also be advantageous when protic acids lead to unwanted side reactions.
Ultimately, the choice of deprotection reagent requires a thorough understanding of the substrate's properties and the reaction conditions. Careful experimentation and optimization are often necessary to achieve the desired result.
The selection of the most appropriate deprotection reagent is, of course, critical, but understanding how the deprotection reaction actually proceeds is equally important for ensuring efficient and successful removal of the Boc group. Grasping the underlying mechanism allows for informed decisions regarding reaction conditions, solvent choice, and troubleshooting potential issues, ultimately leading to higher yields and purer products.
Understanding the Reaction Mechanism for Efficient Deprotection
A firm grasp of the Boc deprotection mechanism is essential for optimizing reaction efficiency. By understanding each step, chemists can make informed decisions about solvent selection, temperature, and reaction time, all of which significantly impact the success of the deprotection.
Detailed Explanation of the Reaction Mechanism
The Boc deprotection mechanism typically involves a two-step process initiated by protonation of the carbonyl oxygen of the Boc group. This protonation is usually carried out by a strong acid, such as TFA or HCl, although the exact acid can vary.
This protonation increases the electrophilicity of the carbonyl carbon, making it more susceptible to nucleophilic attack.
Next, the tert-butyl carbamate undergoes cleavage. The protonated Boc group breaks down into isobutylene, carbon dioxide, and the deprotected amine.
The isobutylene is typically scavenged to prevent unwanted side reactions such as alkylation of the deprotected amine. Carbon dioxide, being a gas, is easily removed from the reaction mixture.
The exact mechanism can vary slightly depending on the specific acid and reaction conditions employed, but the fundamental principles of protonation and cleavage remain the same.
The Role of Solvents
The solvent plays a critical role in facilitating the Boc deprotection reaction. It influences the rate of the reaction, the stability of the intermediates, and the solubility of the reactants and products.
Common Solvents and Their Influence
Dichloromethane (DCM) is a common solvent for Boc deprotections due to its ability to dissolve a wide range of organic compounds. It’s also relatively inert and easy to remove in vacuo.
Other solvents, such as ethyl acetate or acetonitrile, can also be used depending on the solubility of the starting material and the desired reaction conditions.
The choice of solvent can also affect the selectivity of the deprotection. For example, some solvents may favor the formation of certain byproducts over others.
Solvent Polarity and Reaction Rate
Solvent polarity is a key factor influencing the rate of the Boc deprotection reaction. Polar protic solvents, such as alcohols, can stabilize the protonated Boc group, which can accelerate the reaction. However, protic solvents can also protonate the amine, which might generate unwanted side-reactions.
Conversely, nonpolar aprotic solvents, such as DCM, may not stabilize the protonated Boc group as effectively, leading to slower reaction rates.
The ideal solvent polarity will depend on the specific reaction conditions and the nature of the substrate.
Optimizing Reaction Temperature and Time
Reaction temperature and time are critical parameters that must be carefully optimized for efficient Boc deprotection. Increasing the reaction temperature can accelerate the rate of the reaction, but it can also lead to the formation of unwanted byproducts.
In general, Boc deprotections are carried out at temperatures ranging from 0 °C to room temperature. The optimal temperature will depend on the specific acid and solvent employed, as well as the stability of the substrate.
Reaction time must also be carefully controlled. If the reaction is allowed to proceed for too long, it can lead to the formation of byproducts. Conversely, if the reaction is stopped too soon, it can result in incomplete deprotection.
The optimal reaction time will depend on the reaction temperature, the concentration of the acid, and the nature of the substrate. Monitoring the reaction progress using techniques such as thin-layer chromatography (TLC) or HPLC is highly recommended.
The exact mechanism can vary slightly depending on the specific acid and reaction conditions employed, but the fundamental principles of protonation and cleavage remain the same.
Having a solid grasp of the mechanism at play allows us to anticipate potential pitfalls. With this knowledge, we can be proactive in addressing issues that may arise, such as unwanted side reactions.
Tackling Side Reactions and Utilizing Scavengers
Boc deprotection, while generally efficient, isn't always a clean, straightforward process. Several side reactions can occur, impacting the yield and purity of the desired deprotected amine.
These side reactions often stem from the highly reactive intermediates formed during the deprotection process or from the deprotection reagent itself. Understanding these potential issues is crucial for mitigating their effects and achieving optimal results.
Identifying Potential Side Reactions
One common side reaction is the alkylation of the deprotected amine. Isobutylene, a byproduct of Boc cleavage, is a reactive alkene that can alkylate the newly formed amine.
This is particularly problematic with primary amines, which are more nucleophilic and thus more susceptible to alkylation.
Another potential issue is the formation of t-butyl esters if carboxylic acids are present in the reaction mixture. The acidic conditions of the deprotection can lead to esterification, resulting in unwanted byproducts.
Additionally, oligomerization or polymerization of isobutylene can occur, particularly at higher temperatures or concentrations of acid. These polymeric byproducts can be difficult to remove from the desired product.
Finally, the deprotection reagent itself can contribute to side reactions. For example, TFA can react with sensitive functional groups, leading to trifluoroacetylation. This is less common, but it's a possibility that needs to be considered, especially when dealing with complex molecules.
The Consequences of Untreated Side Reactions
The consequences of these side reactions can be significant. Alkylation, esterification, or trifluoroacetylation can lead to lower yields of the desired deprotected amine.
The presence of unwanted byproducts also complicates purification, often requiring extensive chromatography or other separation techniques. In severe cases, side reactions can render the reaction useless, requiring a complete restart.
Therefore, proactive measures to prevent or minimize these side reactions are essential for ensuring the success of the Boc deprotection.
The Necessity and Role of Scavengers
Scavengers are additives used to trap or react with reactive intermediates, preventing them from participating in unwanted side reactions. They are an indispensable tool in Boc deprotection, particularly when dealing with sensitive substrates or when high purity is required.
By selectively reacting with problematic byproducts like isobutylene, scavengers effectively shift the equilibrium towards the desired deprotection pathway, improving yield and simplifying purification.
The choice of scavenger depends on the specific side reaction being targeted and the compatibility with the reaction conditions.
Commonly Used Scavengers and Their Mechanisms
Several scavengers are commonly used in Boc deprotection, each with its own mechanism of action.
-
Triethylsilane (TES): TES is a popular scavenger for trapping isobutylene. It reacts with isobutylene via hydride transfer, converting it into isobutane, a relatively inert gas that is easily removed from the reaction mixture. TES is particularly effective in preventing alkylation of the deprotected amine.
-
Thioanisole: Thioanisole acts as a scavenger by reacting with electrophilic species, such as t-butyl cations formed during Boc cleavage. This prevents the t-butyl group from reacting with other nucleophilic sites in the molecule, such as carboxylic acids.
-
Water: While it might seem counterintuitive, adding small amounts of water can act as a scavenger. Water can react with isobutylene to form t-butanol, which is less reactive towards amines than isobutylene itself.
-
Other Scavengers: Other scavengers include phenol, indole, and various thiols. The choice of scavenger depends on the specific reaction conditions and the potential side reactions of concern.
By carefully selecting and employing the appropriate scavenger, chemists can significantly improve the efficiency and reliability of Boc deprotection reactions. Scavengers are crucial for mitigating side reactions, ensuring high yields, and simplifying the purification of valuable target molecules.
Tackling side reactions and employing scavengers are essential to maintaining the integrity of the deprotection process. But what does a well-executed Boc deprotection look like in practice?
Step-by-Step Guide to Performing Boc Deprotection
This section provides a practical, step-by-step guide to performing a typical Boc deprotection reaction. It covers the reaction procedure itself, as well as the crucial workup and purification techniques necessary to isolate your desired product in high yield and purity.
Detailed Deprotection Procedure
-
Preparation: Begin by dissolving your Boc-protected amine (or amino acid derivative) in a suitable solvent. Dichloromethane (DCM) is a frequently used solvent due to its inertness and ability to dissolve many organic compounds.
The concentration of the substrate typically ranges from 0.1 M to 1.0 M, depending on the scale of the reaction and the solubility of the starting material.
-
Cooling (Optional): If the deprotection is known to be exothermic, consider cooling the reaction mixture to 0 °C (ice bath) before adding the deprotecting agent. This can help to minimize side reactions.
-
Addition of Deprotecting Agent: Slowly add the chosen deprotecting agent to the solution.
- TFA: Trifluoroacetic acid (TFA) is often used neat (without dilution) or as a solution in DCM. A typical concentration of TFA in DCM is 20-50% (v/v).
- HCl: Hydrochloric acid (HCl) is commonly used as a solution in dioxane (e.g., 4M HCl in dioxane). The molar excess of the deprotecting agent usually ranges from 2 to 10 equivalents, depending on the substrate and reaction conditions.
Add the acid dropwise with stirring.
-
Reaction Monitoring: Allow the reaction to proceed under stirring at room temperature.
Monitor the progress of the deprotection using Thin Layer Chromatography (TLC) or High-Performance Liquid Chromatography (HPLC). The reaction time can vary from 30 minutes to several hours, depending on the substrate and the deprotecting agent.
-
Quenching: Once the reaction is complete, quench the reaction by carefully adding a base to neutralize the acidic solution.
Use saturated sodium bicarbonate solution, added slowly until the solution reaches a neutral pH (pH ~7).
Workup and Purification Techniques
Following the deprotection, proper workup and purification are essential to remove residual reagents, byproducts, and scavengers, leading to a pure product.
Neutralization, Extraction, and Washing
-
Neutralization: As described above, neutralize the reaction mixture with a base, carefully monitoring the pH. This step is critical to prevent decomposition of the deprotected amine and to facilitate extraction.
-
Extraction: Extract the aqueous layer with an organic solvent (e.g., ethyl acetate or DCM) to isolate the product. Perform multiple extractions to ensure maximum recovery of the product. Combine the organic layers.
-
Washing: Wash the combined organic layers with water and brine (saturated sodium chloride solution). The water wash removes any remaining salts or polar impurities, while the brine wash helps to remove excess water from the organic layer.
-
Drying: Dry the organic layer over a drying agent such as anhydrous sodium sulfate or magnesium sulfate. This removes any traces of water that could interfere with subsequent purification steps.
-
Filtration and Evaporation: Filter the dried organic layer to remove the drying agent, and then evaporate the solvent under reduced pressure using a rotary evaporator. This will yield the crude deprotected amine.
Purification Methods
If the crude product is not sufficiently pure, further purification is necessary.
-
Recrystallization: If the deprotected amine is a solid, recrystallization can be an effective method for purification. Dissolve the crude product in a minimum amount of hot solvent, then allow the solution to cool slowly.
The pure product will crystallize out of the solution, leaving impurities behind. Filter the crystals and dry them to obtain the purified amine.
-
Chromatography: Chromatography is a powerful technique for separating and purifying organic compounds.
-
Flash Column Chromatography: A common method for purifying organic compounds. The crude product is loaded onto a column packed with silica gel or alumina, and then eluted with a solvent or a mixture of solvents. The different components of the mixture will elute at different rates, allowing for their separation.
Careful selection of the eluent system is critical for achieving good separation.
-
High-Performance Liquid Chromatography (HPLC): Can be used for both analytical and preparative purposes. In preparative HPLC, larger quantities of the crude product are injected onto the column, and the separated fractions are collected.
HPLC is particularly useful for purifying compounds that are difficult to separate by other methods.
-
Tackling side reactions and employing scavengers are essential to maintaining the integrity of the deprotection process. But what does a well-executed Boc deprotection look like in practice? Once the reaction is complete, how do you definitively know that the protecting group has been successfully removed and that you've obtained your desired product?
Analyzing Your Results: Confirmation Techniques
After performing Boc deprotection, it's crucial to confirm the successful removal of the protecting group and the formation of the desired product. Analytical techniques are indispensable tools for this purpose. Among the most commonly employed techniques are Nuclear Magnetic Resonance (NMR) spectroscopy and Mass Spectrometry (MS).
NMR Spectroscopy for Deprotection Confirmation
NMR spectroscopy is a powerful technique that provides detailed information about the structure and environment of molecules. By analyzing the NMR spectrum of your product, you can determine whether the Boc group has been successfully removed.
Key NMR Signals to Monitor
-
Disappearance of Boc-Specific Signals: The most direct evidence of successful deprotection is the disappearance of signals characteristic of the Boc group.
- Specifically, look for the absence of the tert-butyl peak (typically around 1.4-1.5 ppm in ¹H NMR) and the carbonyl carbon peak (around 150-155 ppm in ¹³C NMR).
-
Appearance of New Signals: Deprotection often leads to the appearance of new signals or changes in the chemical shifts of existing signals. For example, if you are deprotecting an amine, the protons on the amine nitrogen may become more visible and their chemical shift may change.
-
Changes in Coupling Patterns: Deprotection can also alter coupling patterns in the NMR spectrum. Analyzing these changes can provide additional confirmation of successful deprotection.
Sample Preparation and Spectral Interpretation
Proper sample preparation is essential for obtaining high-quality NMR spectra. Use a suitable deuterated solvent (e.g., CDCl₃, DMSO-d₆) and ensure that the sample is fully dissolved.
Carefully interpret the NMR spectrum, paying attention to chemical shifts, peak intensities, and coupling patterns. Compare the spectrum of your product with that of the starting material to identify the changes that occurred during deprotection.
Mass Spectrometry for Deprotection Confirmation
Mass spectrometry is another valuable technique for confirming Boc deprotection. MS provides information about the mass-to-charge ratio (m/z) of ions, allowing you to determine the molecular weight of your product.
Identifying the Deprotected Product
-
Expected Mass Shift: The most straightforward way to confirm deprotection using MS is to look for the expected mass shift. The Boc group has a molecular weight of 100.12 g/mol (C₅H₉O₂). Successful deprotection should result in a decrease of 100.12 Da in the mass of the product.
-
Fragmentation Patterns: In addition to the molecular ion peak, MS also provides information about the fragmentation patterns of the molecule. Analyzing these patterns can provide additional confirmation of the product's identity.
Ionization Techniques and Data Analysis
Various ionization techniques can be used for MS analysis, including electrospray ionization (ESI) and matrix-assisted laser desorption/ionization (MALDI).
Select the appropriate ionization technique based on the properties of your product. Analyze the MS data carefully, paying attention to the mass-to-charge ratios of the ions and their relative abundances.
By combining NMR spectroscopy and mass spectrometry, you can obtain a comprehensive picture of your product's structure and purity, providing strong evidence of successful Boc deprotection. These techniques, when used in conjunction, significantly bolster the confidence in the outcome of your reaction.
Safety First: Handling Deprotection Reagents with Care
The successful execution of Boc deprotection hinges not only on a deep understanding of chemical principles but also on a stringent commitment to safety. Deprotection reagents, particularly strong acids like TFA and HCl, pose significant hazards if not handled with meticulous care. Prioritizing safety is not merely a regulatory obligation; it is a fundamental ethical responsibility for all chemists.
Essential Personal Protective Equipment (PPE)
The first line of defense against chemical exposure lies in the consistent and correct use of Personal Protective Equipment (PPE). This includes, but is not limited to:
-
Gloves: Chemical-resistant gloves, such as nitrile or neoprene gloves, are crucial for preventing skin contact with corrosive reagents. The selection of glove material should be based on the specific chemicals being used and the duration of exposure. Always check a glove compatibility chart to ensure the gloves will provide adequate protection. Damaged or compromised gloves should be replaced immediately.
-
Eye Protection: Safety goggles or a face shield are absolutely essential to protect the eyes from splashes or fumes. Contact lenses should be avoided, as they can trap chemicals against the eye in case of an accident and exacerbate the damage.
-
Lab Coat: A chemically resistant lab coat provides a barrier between your clothing and potential spills. Lab coats should be buttoned up and worn at all times in the laboratory.
-
Closed-Toe Shoes: Shoes that fully cover the feet are mandatory in the lab. Sandals or open-toe shoes offer no protection against chemical spills or dropped objects.
The Importance of a Well-Ventilated Workspace
Many deprotection reactions release volatile and potentially harmful fumes. Performing these reactions in a well-ventilated area, such as a properly functioning fume hood, is critical to minimize inhalation exposure.
Ensure the fume hood is inspected regularly and that the airflow is sufficient to effectively remove vapors from the breathing zone. Avoid placing your head inside the fume hood, and keep the sash at the recommended height to maintain optimal airflow. If a fume hood is unavailable, consider using a portable ventilation system or performing the reaction in an open area with adequate airflow, although this is generally less desirable.
Managing Chemical Waste: A Responsible Approach
Proper waste disposal is an integral component of safe laboratory practices. Never dispose of chemical waste down the drain unless explicitly permitted by your institution's guidelines.
Segregate waste streams based on chemical compatibility and hazard classification. Acidic waste should be collected separately from organic waste, and halogenated solvents should be segregated from non-halogenated solvents.
Ensure all waste containers are clearly labeled with their contents and hazard warnings. Follow your institution's specific waste disposal procedures, which may involve neutralization, solvent recovery, or disposal through a licensed waste disposal company. Familiarize yourself with local, state, and federal regulations regarding chemical waste disposal.
Responding to Spills and Accidental Exposure
Despite the best precautions, accidents can still occur. It's essential to be prepared to respond quickly and effectively to chemical spills and accidental exposure.
-
Spills: Have spill cleanup materials readily available, such as absorbent pads, neutralizing agents, and appropriate personal protective equipment. Contain the spill immediately to prevent it from spreading. Neutralize acids with a suitable base, and absorb the spilled material with absorbent pads. Dispose of the contaminated materials according to your institution's waste disposal procedures.
-
Skin Contact: If a chemical comes into contact with your skin, immediately flush the affected area with copious amounts of water for at least 15 minutes. Remove any contaminated clothing. Seek medical attention if irritation persists or if the chemical is particularly hazardous.
-
Eye Contact: If a chemical enters your eyes, immediately flush them with water for at least 15 minutes, lifting the upper and lower eyelids to ensure thorough rinsing. Seek immediate medical attention.
-
Inhalation: If you inhale chemical fumes, move to fresh air immediately. Seek medical attention if you experience any respiratory distress.
Cultivating a Culture of Safety
Ultimately, safety is not just a set of rules and procedures, but a mindset. It requires a proactive approach, with every chemist taking personal responsibility for their own safety and the safety of those around them. Encourage open communication about safety concerns, and foster a culture where reporting near misses and accidents is encouraged, not discouraged. Regular safety training, hazard assessments, and adherence to standard operating procedures are essential for maintaining a safe and productive laboratory environment.
Specific Applications: Boc Deprotection in Peptide Synthesis
Having established a foundation for the safe and effective handling of deprotection reagents, let's now pivot to a crucial application of Boc deprotection: peptide synthesis. This field relies heavily on the controlled removal of protecting groups to sequentially couple amino acids, ultimately building complex peptide chains.
The strategic use of Boc as a temporary protecting group for the alpha-amino group of amino acids is particularly prominent, especially in classical solution-phase peptide synthesis. However, it also sees significant use, albeit with certain caveats, in solid-phase peptide synthesis (SPPS).
Boc Deprotection in Peptide Chemistry: A Closer Look
The use of Boc-protected amino acids offers several advantages in peptide synthesis. The Boc group is readily removed under relatively mild acidic conditions, typically using trifluoroacetic acid (TFA) in dichloromethane (DCM). This selectivity is crucial, as it allows for the deprotection of the alpha-amino group without cleaving other acid-labile protecting groups that may be present on amino acid side chains.
Furthermore, the byproducts of Boc deprotection, isobutylene and carbon dioxide, are volatile and easily removed from the reaction mixture, simplifying purification processes.
However, the acidic conditions required for Boc deprotection can also lead to unwanted side reactions, such as the alkylation of sensitive amino acid side chains or the cleavage of acid-labile linkages within the peptide sequence. Therefore, careful optimization of the reaction conditions, including the concentration of TFA, the reaction time, and the temperature, is essential to minimize these side reactions and maximize the yield and purity of the desired peptide.
Considerations for Solution-Phase Peptide Synthesis
In solution-phase peptide synthesis, Boc-protected amino acids are typically coupled using activating agents like dicyclohexylcarbodiimide (DCC) or benzotriazol-1-yl-oxytripyrrolidinophosphonium hexafluorophosphate (PyBOP). After each coupling step, the Boc group is removed to reveal the free amino group, which can then be coupled to the next amino acid.
This iterative process requires careful monitoring and purification at each step to ensure the formation of a homogeneous product.
The choice of solvent is also critical in solution-phase peptide synthesis. Solvents like dimethylformamide (DMF) and dimethylacetamide (DMA) are commonly used due to their ability to dissolve both the protected amino acids and the growing peptide chain. However, these solvents can also promote side reactions, so it is important to use them in anhydrous form and to minimize the reaction time.
Special Considerations for Solid-Phase Peptide Synthesis (SPPS)
While Fmoc chemistry has become the dominant strategy in SPPS, Boc chemistry still holds a niche. In Boc-based SPPS, the C-terminal amino acid is attached to a solid support, and the peptide chain is elongated from the C-terminus to the N-terminus. The Boc group is used to protect the alpha-amino group of each incoming amino acid.
Acid Lability and Resin Choice
A key difference between Boc- and Fmoc-based SPPS lies in the acid lability of the protecting groups and the linkage to the resin. Boc deprotection requires strong acidic conditions (typically TFA), which can also cleave the peptide from the resin. Therefore, resins used in Boc-SPPS are typically more acid-stable than those used in Fmoc-SPPS.
Scavengers in Boc-SPPS
Due to the harsher acidic conditions of Boc deprotection in SPPS, the use of scavengers is particularly important. Scavengers such as triisopropylsilane (TIPS), ethanedithiol (EDT), and thioanisole are added to the deprotection cocktail to trap reactive carbocations that can lead to side reactions, such as alkylation of the peptide or modification of amino acid side chains.
Advantages and Disadvantages of Boc-SPPS
While Fmoc-SPPS is generally preferred due to its milder reaction conditions, Boc-SPPS can offer some advantages in certain situations. For example, Boc-SPPS may be more suitable for the synthesis of peptides containing acid-sensitive modifications or for the synthesis of peptides on a large scale. However, the potential for side reactions and the need for careful optimization make Boc-SPPS a more challenging technique than Fmoc-SPPS.
In conclusion, Boc deprotection plays a crucial role in peptide synthesis, enabling the controlled and selective removal of the Boc protecting group to facilitate the formation of peptide bonds. While the acidic conditions required for Boc deprotection can pose challenges, careful optimization of the reaction conditions and the use of appropriate scavengers can minimize side reactions and maximize the yield and purity of the desired peptide. Understanding these nuances is vital for any chemist undertaking peptide synthesis, whether in solution or on a solid support.
Troubleshooting Common Issues and Optimizing Your Reaction
Even with a solid understanding of the Boc deprotection mechanism and the right reagents at hand, challenges can arise. Recognizing common pitfalls and implementing optimization strategies are essential for achieving consistently high yields and purity in your reactions. This section provides practical advice on troubleshooting problems and fine-tuning your deprotection protocol.
Common Problems Encountered During Boc Deprotection
Several issues can plague Boc deprotection reactions, impacting yield, purity, and overall success. Understanding these problems is the first step towards resolving them.
Incomplete Deprotection
Incomplete deprotection is a frequent issue, leaving some of the starting material unreacted. Several factors can contribute, including:
-
Insufficient reaction time: Ensure the reaction proceeds for a sufficient duration.
-
Low reagent concentration: Increase the concentration of the deprotection reagent.
-
Low reaction temperature: Increasing the temperature may help, but be mindful of potential side reactions.
-
Steric hindrance: Bulky substituents near the Boc group can hinder deprotection. Consider using a more potent deprotection reagent.
Side Reactions and Byproduct Formation
The acidic conditions required for Boc deprotection can promote unwanted side reactions, particularly alkylation of sensitive amino acid side chains or cleavage of acid-labile protecting groups.
Scavengers are crucial for mitigating these side reactions.
Common byproducts can also arise from the deprotection reagent itself. For example, TFA can react with certain compounds to form trifluoroacetylated products. Using appropriate scavengers and controlling the reaction conditions can minimize these issues.
Product Degradation
The target molecule itself can be susceptible to degradation under the acidic conditions of Boc deprotection.
This is more common with complex or sensitive molecules.
-
Reducing the reaction time.
-
Lowering the reaction temperature.
-
Using milder deprotection conditions.
-
Carefully selecting the appropriate scavenger.
Can help minimize degradation.
Solubility Issues
The starting material, product, or both may exhibit poor solubility in the reaction solvent. Poor solubility can significantly hinder the reaction rate and yield.
- Experiment with different solvents or solvent mixtures to improve solubility.
- Adding a co-solvent, such as methanol or acetonitrile, can sometimes improve solubility without interfering with the reaction.
Difficulties in Workup and Purification
The deprotection reaction mixture can be complex, containing the desired product, unreacted starting material, byproducts, and the deprotection reagent itself. Efficient workup and purification are essential for isolating the pure product.
- Optimize the extraction and washing steps to remove impurities selectively.
- Choose a purification method appropriate for the specific molecule, such as chromatography (e.g., flash chromatography, HPLC) or crystallization.
Tips and Tricks for Reaction Optimization
Optimizing the Boc deprotection reaction involves carefully adjusting the reaction conditions to maximize yield, minimize side reactions, and simplify the workup and purification process.
Reagent Selection and Concentration
The choice of deprotection reagent (TFA, HCl, etc.) and its concentration significantly impact the reaction outcome.
- TFA is a common choice, but HCl may be preferred for substrates sensitive to TFA.
- Optimize the reagent concentration to achieve complete deprotection without promoting excessive side reactions. Titration can be helpful to ensure the solution is at the proper molarity.
Solvent Effects
The solvent plays a critical role in the reaction.
- Dichloromethane (DCM) is a common solvent for Boc deprotection, but other solvents, such as ethyl acetate or acetonitrile, may be suitable depending on the substrate's solubility and reactivity.
- Ensure the solvent is dry and free of impurities that could interfere with the reaction.
Temperature Control
The reaction temperature significantly affects the reaction rate and selectivity.
- Lower temperatures can minimize side reactions but may also slow down the deprotection.
- Higher temperatures can accelerate the reaction but may increase the risk of product degradation.
- Carefully control the temperature using an ice bath or a heating mantle to maintain the desired reaction temperature.
Reaction Time
The reaction time must be optimized to ensure complete deprotection without overexposing the product to acidic conditions.
- Monitor the reaction progress using TLC or HPLC to determine the optimal reaction time.
- Avoid excessively long reaction times, as this can increase the risk of side reactions and product degradation.
Scavenger Selection and Usage
Scavengers are essential for trapping reactive intermediates and preventing side reactions.
- Triethylsilane (TES) is a common scavenger for carbocations.
- Water can act as a scavenger for isobutylene.
- The choice of scavenger depends on the specific side reactions that are likely to occur.
- Optimize the amount of scavenger to effectively trap reactive intermediates without interfering with the desired reaction.
Monitoring Reaction Progress
Monitoring the reaction progress is crucial for determining the optimal reaction time and identifying any problems that may arise.
- Thin-layer chromatography (TLC) is a simple and convenient method for monitoring the disappearance of the starting material and the appearance of the product.
- High-performance liquid chromatography (HPLC) provides more quantitative information about the reaction progress.
- NMR spectroscopy can be used to identify and quantify the starting material, product, and any byproducts.
Workup and Purification Strategies
Efficient workup and purification are essential for isolating the pure product after deprotection.
- Extraction can be used to remove the deprotection reagent and any water-soluble impurities.
- Washing the organic layer with a base can remove any remaining acid.
- Chromatography (e.g., flash chromatography, HPLC) is often necessary to purify the product further.
- Crystallization can be used to obtain a highly pure product if the compound is crystalline.
By systematically addressing these common problems and implementing appropriate optimization strategies, you can significantly improve the yield and purity of your Boc deprotection reactions and achieve consistently successful results.
Video: Boc Deprotection: The Expert's Complete How-To Guide
Frequently Asked Questions About Boc Deprotection
Here are some common questions about Boc deprotection to help clarify the process and its nuances.
What exactly is Boc deprotection?
Boc deprotection is a chemical reaction that removes the Boc (tert-butoxycarbonyl) protecting group. This group is commonly used to protect amines in organic synthesis. Removing the Boc group exposes the amine for further reactions.
Why is Boc deprotection necessary?
The Boc group is a protecting group, meaning it temporarily blocks the amine's reactivity. Boc deprotection is necessary to remove this protecting group, allowing the amine to participate in subsequent chemical reactions to build more complex molecules.
What are the common reagents used for Boc deprotection?
The most common reagents include strong acids like trifluoroacetic acid (TFA) or hydrochloric acid (HCl). These acids protonate the Boc group, leading to its cleavage and liberation of the amine.
Can Boc deprotection damage other parts of my molecule?
Yes, using harsh conditions can lead to unwanted side reactions. It's crucial to choose the appropriate deprotection method and conditions based on the other functional groups present in your molecule. Milder reagents or alternative protecting groups might be necessary in certain cases to minimize side reactions during Boc deprotection.
So there you have it! Hopefully, this guide cleared up any confusion around boc deprotection. Now, go forth and synthesize! Good luck with your experiments!