Benzoic Acid IR Spectrum: Demystified for Easy Understanding
Infrared Spectroscopy, a powerful analytical technique, provides insights into the vibrational modes of molecules, making it invaluable for compound identification. Benzoic acid, a common aromatic carboxylic acid, exhibits a distinctive benzoic acid IR spectrum. The interpretation of a spectrum such as the benzoic acid IR spectrum often requires comparison against spectral databases, allowing for confident confirmation of a compound's presence and purity. Understanding the relationship between benzoic acid's structure and its benzoic acid IR spectrum is therefore crucial for many applications.
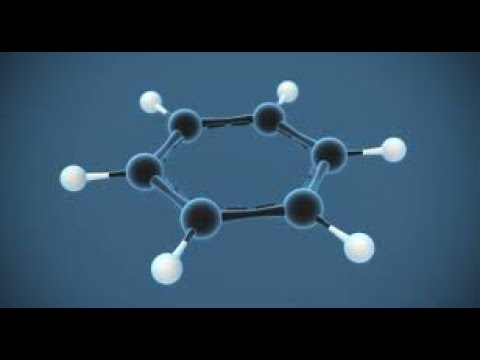
Image taken from the YouTube channel IB Chemistry Help , from the video titled IB Chemistry - Benzene ring, benzoic acid structure and IR spectrum explained! .
Benzoic acid, a seemingly simple aromatic carboxylic acid, plays an surprisingly versatile role in a vast range of applications, from food preservation to pharmaceutical synthesis. Understanding its unique properties is crucial for chemists, biologists, and material scientists alike.
Infrared (IR) spectroscopy offers a powerful method for identifying and characterizing molecules by analyzing their vibrational modes. Each molecule possesses a unique IR spectrum, acting as a molecular fingerprint that reveals its structural composition and characteristics.
This article aims to provide a clear and accessible explanation of the benzoic acid IR spectrum. It is designed for both newcomers to spectroscopy and those with prior experience seeking a deeper understanding of this important compound. By dissecting the spectrum, we will uncover the relationship between benzoic acid's structure and its characteristic infrared absorption patterns.
Benzoic Acid: A Ubiquitous Compound
Benzoic acid (C6H5COOH) is a colorless, crystalline solid found naturally in many plants and fruits.
Its significance stems from its diverse applications:
-
As a food preservative, it inhibits the growth of bacteria, yeast, and molds.
-
In the pharmaceutical industry, it serves as a key intermediate in the synthesis of numerous drugs.
-
Industrially, it is used in the production of plastics, resins, and dyes.
Its widespread use underscores the importance of reliable methods for its identification and quality control, where IR spectroscopy excels.
The Power of IR Spectroscopy
IR spectroscopy operates on the principle that molecules absorb infrared radiation at specific frequencies that correspond to the vibrational modes of their bonds.
When a molecule absorbs IR radiation, it undergoes vibrational excitation. These vibrations include:
-
Stretching: Changes in bond length.
-
Bending: Changes in bond angle.
Each vibrational mode absorbs energy at a characteristic frequency, measured as wavenumber (cm-1).
By analyzing the pattern of absorption bands in an IR spectrum, we can identify the functional groups present in the molecule and gain insights into its structure. This makes IR spectroscopy an indispensable tool for:
-
Identifying unknown substances.
-
Verifying the purity of compounds.
-
Studying molecular structure and dynamics.
Decoding the Benzoic Acid Spectrum: Purpose and Scope
This article offers a comprehensive guide to interpreting the IR spectrum of benzoic acid. We will delve into the key spectral regions:
- The carbonyl (C=O) region.
- The hydroxyl (O-H) region.
- The aromatic ring region.
We will analyze the characteristic peaks associated with each functional group, explaining their positions, intensities, and shapes. Factors that can influence the spectrum, such as hydrogen bonding, will also be discussed.
By the end of this article, you will have a solid understanding of the benzoic acid IR spectrum and the ability to use it for identification, quality control, and structural elucidation. We aim to equip you with the knowledge and confidence to interpret this vital molecular fingerprint.
The Theoretical Foundation: How IR Spectroscopy Works
Having established benzoic acid's significance and IR spectroscopy's utility, it's crucial to delve into the fundamental principles that govern this powerful analytical technique. Understanding these principles allows for a more informed and nuanced interpretation of IR spectra, moving beyond simple peak identification to a deeper appreciation of the molecular dynamics at play.
Molecular Vibrations and IR Absorption
At its core, IR spectroscopy hinges on the interaction between infrared radiation and the vibrational modes of molecules. Molecules are not static entities; their atoms are constantly in motion, vibrating around their equilibrium positions.
These vibrations can be categorized into various modes, such as stretching (changes in bond length) and bending (changes in bond angle). Each vibrational mode has a specific frequency associated with it.
When a molecule is irradiated with infrared light, it will absorb energy if the frequency of the radiation matches the frequency of a particular vibrational mode. This absorption of energy causes an increase in the amplitude of that vibration.
However, not all vibrations are IR active. For a molecule to absorb IR radiation, the vibration must cause a change in the dipole moment of the molecule. This means that symmetrical molecules or vibrations that do not result in a change in charge distribution will not absorb IR light.
Wavenumber: The Spectroscopic Yardstick
IR spectra are typically plotted as transmittance or absorbance versus wavenumber. Wavenumber (ν̃), expressed in units of inverse centimeters (cm-1), is the number of wavelengths per unit distance. It is directly proportional to the frequency and energy of the IR radiation.
Wavenumber is preferred over wavelength (λ) because it is linearly proportional to energy, making it more convenient for correlating spectral features with molecular vibrations. A higher wavenumber corresponds to a higher frequency and thus higher energy vibration.
The relationship between wavenumber, frequency (ν), and the speed of light (c) is given by:
ν̃ = ν / c
This means that a peak at 1700 cm-1 represents an absorption of IR radiation with a specific frequency and energy related to a particular molecular vibration.
Functional Groups and Absorption Bands
Functional groups are specific arrangements of atoms within a molecule that exhibit characteristic chemical behaviors. They also give rise to characteristic absorption bands in the IR spectrum.
The position (wavenumber), intensity, and shape of these bands can provide valuable information about the presence and environment of specific functional groups within a molecule.
For example, carbonyl groups (C=O) typically exhibit strong absorption bands in the range of 1650-1800 cm-1, while hydroxyl groups (O-H) often show broad absorptions in the range of 2500-3300 cm-1 due to hydrogen bonding.
By analyzing the positions and characteristics of these absorption bands, one can identify the functional groups present in a molecule and gain insights into its structure and properties.
Some common functional groups and their expected wavenumber ranges are shown below:
- Alkanes (C-H stretch): 2850-3000 cm-1
- Alkenes (C=C stretch): 1620-1680 cm-1
- Aromatic Rings (C=C stretch): 1450-1600 cm-1
- Alcohols (O-H stretch): 3200-3600 cm-1
- Amines (N-H stretch): 3300-3500 cm-1
Understanding the relationship between functional groups and their corresponding absorption bands is essential for interpreting IR spectra and elucidating the structure of unknown compounds.
Decoding the Benzoic Acid IR Spectrum: A Detailed Analysis
Having established the theoretical underpinnings of IR spectroscopy, we now turn our attention to the practical application of these principles in deciphering the IR spectrum of benzoic acid. This section provides a detailed exploration of the benzoic acid IR spectrum, focusing on key regions and characteristic peaks, with a particular emphasis on the signatures of the carboxylic acid group and the aromatic ring.
An Overview of the Benzoic Acid IR Spectrum
The IR spectrum of benzoic acid presents a rich tapestry of absorption bands, each corresponding to a specific vibrational mode within the molecule. To effectively navigate this spectrum, it's helpful to divide it into key regions: the carbonyl region (approximately 1650-1800 cm-1), the hydroxyl region (typically 2500-3300 cm-1), and the aromatic region (1450-1600 cm-1 and 3000-3100 cm-1).
[Note: A representative spectrum image of benzoic acid should be inserted here to visually aid the reader.]
Each of these regions provides crucial information about the presence and environment of the corresponding functional groups. By carefully analyzing the position, intensity, and shape of the peaks within these regions, we can gain a comprehensive understanding of the molecular structure and bonding characteristics of benzoic acid.
In-Depth Analysis of the Carboxylic Acid Region
The carboxylic acid functional group (-COOH) is the defining feature of benzoic acid, and its spectral signature is particularly informative. This region displays two prominent peaks: one corresponding to the carbonyl (C=O) stretch and another to the hydroxyl (O-H) stretch.
The Carbonyl (C=O) Stretch
The carbonyl stretch is typically observed as a strong, sharp peak around 1700 cm-1.
The precise position of this peak is sensitive to several factors, including conjugation and ring strain. For example, conjugation with the aromatic ring in benzoic acid can slightly lower the wavenumber of the carbonyl absorption compared to a non-conjugated carbonyl group.
Similarly, if the carbonyl group were part of a cyclic system experiencing ring strain, the wavenumber would be affected. The intensity of the C=O stretch is generally high due to the large dipole moment change during the vibration.
The Hydroxyl (O-H) Stretch
The O-H stretch in carboxylic acids is characterized by a broad, intense absorption band spanning the region of 2500-3300 cm-1.
This broadness is a direct consequence of hydrogen bonding, which is prevalent in carboxylic acids due to the ability of the hydroxyl group to both donate and accept hydrogen bonds. The hydrogen bonding network creates a distribution of O-H bond lengths and strengths, resulting in a range of vibrational frequencies and, consequently, a broadened peak.
The characteristic shape of the O-H stretch often overlaps with C-H stretches, making its identification challenging. However, the broadness and intensity of the peak are key indicators of the presence of a carboxylic acid.
FTIR and High-Resolution Spectra
Fourier Transform Infrared (FTIR) spectroscopy is the predominant technique used to acquire high-resolution IR spectra.
FTIR instruments employ an interferometer to modulate the infrared beam, allowing for simultaneous measurement of all frequencies. This results in a spectrum with high signal-to-noise ratio and excellent resolution, enabling the detection of subtle spectral features and facilitating accurate peak identification.
FTIR spectrometers excel in rapidly acquiring high-resolution data, which is critical for complex samples. The digital data format allows for computational processing, such as baseline correction and spectral smoothing, to enhance data quality and facilitate analysis.
Analyzing Other Significant Peaks
Beyond the carboxylic acid region, the benzoic acid IR spectrum exhibits additional peaks that provide valuable structural information. These include C-H stretches and aromatic ring vibrations.
C-H (Aliphatic/Aromatic) Stretches
C-H stretches appear in the region of 3000-3100 cm-1, and it's important to distinguish between aromatic and aliphatic contributions.
Aromatic C-H stretches, arising from the benzene ring, typically appear as sharp peaks slightly above 3000 cm-1. Aliphatic C-H stretches, if present (potentially due to impurities), appear below 3000 cm-1.
Aromatic Ring Vibrations
The aromatic ring in benzoic acid gives rise to several characteristic vibrational modes in the 1450-1600 cm-1 region.
These include ring breathing modes and C=C stretches within the aromatic ring. These peaks are often less intense than the carbonyl and hydroxyl stretches but provide important confirmation of the presence of an aromatic ring. The specific positions and intensities of these peaks can be used to identify the substitution pattern on the aromatic ring.
Factors Influencing the Benzoic Acid IR Spectrum and Data Interpretation
Having meticulously examined the key spectral regions characteristic of benzoic acid, we now pivot to a crucial aspect of IR spectroscopy: understanding the factors that can subtly alter the spectrum and refining our approach to data interpretation. These factors, if overlooked, can lead to misinterpretations and inaccurate conclusions. Therefore, a nuanced understanding of these influences is paramount for deriving meaningful insights from the benzoic acid IR spectrum.
The Pervasive Influence of Hydrogen Bonding
Hydrogen bonding, a potent intermolecular force, exerts a significant influence on the IR spectrum of benzoic acid, particularly in the hydroxyl region. The carboxylic acid group (-COOH) in benzoic acid is prone to forming hydrogen bonds with neighboring molecules, leading to a broadening and shifting of the O-H stretch.
This broadening occurs because hydrogen bonding introduces a range of slightly different vibrational energies for the O-H bond.
Instead of a sharp, well-defined peak, we observe a broad absorption band typically spanning the region from approximately 2500 to 3300 cm-1.
The strength and extent of hydrogen bonding are also concentration-dependent; higher concentrations generally favor increased hydrogen bonding and a more pronounced broadening effect. Moreover, the shape of the O-H stretch can offer clues about the nature of hydrogen bonding (e.g., whether it is primarily intermolecular or intramolecular).
Accurate Peak Identification: The Cornerstone of Reliable Analysis
The accuracy of any IR spectral interpretation hinges upon the correct identification of peaks. While spectral databases and literature values provide valuable guidance, relying solely on these resources can be misleading.
A rigorous approach to peak identification requires careful consideration of multiple factors: position, intensity, and shape.
-
Position: Compare the observed wavenumber to the expected range for the functional group in question. Remember that substituents and structural features can shift peak positions slightly.
-
Intensity: Note the relative strength of the absorption. Strong peaks generally correspond to highly polar bonds, while weaker peaks may arise from less polar bonds or lower concentrations.
-
Shape: Pay attention to the peak's shape. Broad peaks often indicate hydrogen bonding or overlapping absorptions, while sharp peaks typically represent well-defined vibrational modes.
Qualitative Analysis: Confirming the Presence of Benzoic Acid
The IR spectrum serves as a molecular fingerprint, allowing for the definitive identification of benzoic acid.
By meticulously comparing the observed spectrum to established reference spectra and characteristic peak positions, one can confidently confirm the presence of benzoic acid in a sample.
The key is to focus on the hallmark peaks associated with the carboxylic acid group and the aromatic ring.
These include the strong carbonyl (C=O) stretch around 1700 cm-1, the broad O-H stretch between 2500-3300 cm-1, and the characteristic aromatic ring vibrations in the 1450-1600 cm-1 and 3000-3100 cm-1 regions. The presence of all these peaks, with appropriate intensities and shapes, provides strong evidence for the identity of benzoic acid.
Data Interpretation Tips: Unlocking the Spectral Secrets
Reading and understanding IR spectral data requires practice and attention to detail. The following tips can help you extract the most valuable information from your benzoic acid IR spectra:
-
Baseline Correction: Ensure that the baseline is properly corrected to remove any sloping or curvature, which can distort peak shapes and intensities. Most spectral software packages offer automated baseline correction tools.
-
Noise Reduction: Spectral noise can obscure weak peaks and make accurate peak identification challenging. Employ noise reduction techniques, such as smoothing, to improve the signal-to-noise ratio. Be cautious not to over-smooth, which can distort genuine spectral features.
-
Careful Examination of Peak Shoulders: Peak shoulders are subtle inflections on the side of a peak that can indicate the presence of overlapping absorptions or subtle structural variations. Carefully examine peak shapes for shoulders and consider their potential origins.
-
Comparison to Reference Spectra: Always compare your obtained spectrum to reliable reference spectra from reputable databases (e.g., NIST) to validate your interpretation and identify any potential impurities.
Having established a robust understanding of the benzoic acid IR spectrum and the factors that can influence it, we now turn our attention to its practical applications. The power of IR spectroscopy lies not only in its ability to identify functional groups, but also in its capacity to provide valuable insights into the identity and purity of benzoic acid samples in diverse real-world scenarios.
Practical Applications and Examples
The benzoic acid IR spectrum is far more than just an academic exercise; it is a powerful tool with numerous applications in research, quality control, and forensic science. By carefully analyzing the IR spectrum, we can glean valuable information about the identity, purity, and even the source of a benzoic acid sample.
Identification of Unknown Samples
One of the most fundamental applications of IR spectroscopy is the identification of unknown substances. If presented with an unknown sample suspected to be benzoic acid, its IR spectrum can provide definitive confirmation.
By comparing the spectrum of the unknown sample to the characteristic peaks we've discussed—the carbonyl stretch around 1700 cm-1, the broad hydroxyl stretch between 2500 and 3300 cm-1, and the aromatic ring vibrations—we can establish whether or not the sample is indeed benzoic acid.
This process involves carefully matching the peak positions, intensities, and shapes to those expected for benzoic acid. Any discrepancies could indicate the presence of impurities or that the substance is not benzoic acid at all.
Purity Assessment
Beyond simple identification, IR spectroscopy can also be used to assess the purity of a benzoic acid sample. In this case, the focus shifts from merely identifying the presence of benzoic acid to quantifying the presence of any contaminants.
The presence of impurities will often manifest as additional peaks in the IR spectrum that are not characteristic of pure benzoic acid. For example, the presence of unreacted starting materials or byproducts from a synthesis might be detected as distinct peaks in the spectrum.
The intensity of these impurity peaks can be correlated to the level of contamination, providing a semi-quantitative measure of the sample's purity. While not as precise as other quantitative analytical techniques, IR spectroscopy offers a rapid and convenient method for assessing purity.
Furthermore, the absence of expected peaks, or changes in their relative intensities, can also signal degradation or alteration of the benzoic acid.
Spectral Comparison with Reference Standards
The true power of IR spectroscopy is unlocked when combined with spectral databases and reference standards. By comparing an obtained spectrum to reference spectra, we can not only confirm the identity of a substance but also gain insights into its purity and even its source.
Leveraging Spectral Databases
Spectral databases, such as those maintained by the National Institute of Standards and Technology (NIST), contain a vast library of IR spectra for a wide range of compounds.
By searching these databases for the benzoic acid spectrum, we can obtain a high-quality reference spectrum against which to compare our own data. This comparison allows for a more rigorous assessment of peak positions, intensities, and shapes, leading to more accurate identification and purity assessment.
Detecting Impurities through Spectral Matching
Moreover, spectral databases can be used to identify potential impurities in a sample. If unexpected peaks are observed in the spectrum, they can be compared to the spectra of known compounds to determine their identity.
This process can be particularly useful in forensic science, where identifying trace amounts of contaminants can provide valuable clues about the origin and history of a sample.
The Value of Reference Standards
In addition to spectral databases, physical reference standards of pure benzoic acid can be invaluable. By running an IR spectrum of a known pure standard, we can ensure that our instrument is properly calibrated and that our data is accurate.
Furthermore, comparing the spectrum of an unknown sample to that of a pure standard allows for a more direct and reliable assessment of purity.
Video: Benzoic Acid IR Spectrum: Demystified for Easy Understanding
Benzoic Acid IR Spectrum: Frequently Asked Questions
[Benzoic acid infrared (IR) spectroscopy can seem daunting, but understanding the key peaks makes it much simpler. Here are some frequently asked questions to help clarify the benzoic acid IR spectrum.]
What are the key peaks to look for in a benzoic acid IR spectrum?
The most important peaks in the benzoic acid IR spectrum are typically around 1680-1725 cm-1 for the C=O stretch of the carboxylic acid, a broad O-H stretch from 2500-3300 cm-1, and C-H stretches in the aromatic ring region (3000-3100 cm-1).
Why is the O-H stretch in the benzoic acid IR spectrum so broad?
The broad O-H stretch in the benzoic acid IR spectrum is due to hydrogen bonding between the carboxylic acid molecules. This intermolecular hydrogen bonding weakens the O-H bond and causes the peak to broaden significantly.
How can I distinguish benzoic acid from a similar aromatic compound using IR spectroscopy?
Focus on the presence of both the carbonyl (C=O) stretch around 1680-1725 cm-1 and the broad O-H stretch between 2500-3300 cm-1. Many aromatic compounds won't exhibit both features in their IR spectrum, making it useful for distinguishing benzoic acid.
What does the region around 3000-3100 cm-1 in the benzoic acid IR spectrum indicate?
The peaks in the 3000-3100 cm-1 region represent the C-H stretches of the aromatic ring in benzoic acid. These peaks are generally sharper than the broad O-H stretch and confirm the presence of an aromatic ring.
So there you have it – a simplified look at the benzoic acid IR spectrum! Hope this helps you make sense of those squiggly lines. Happy analyzing!